Understanding Genetic Engineering: Concepts and Ethics
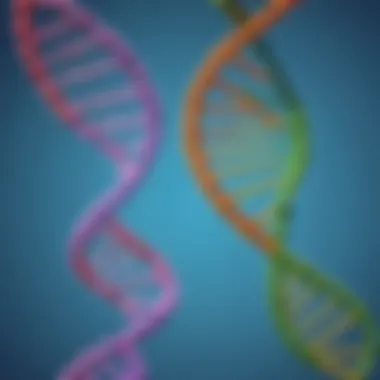
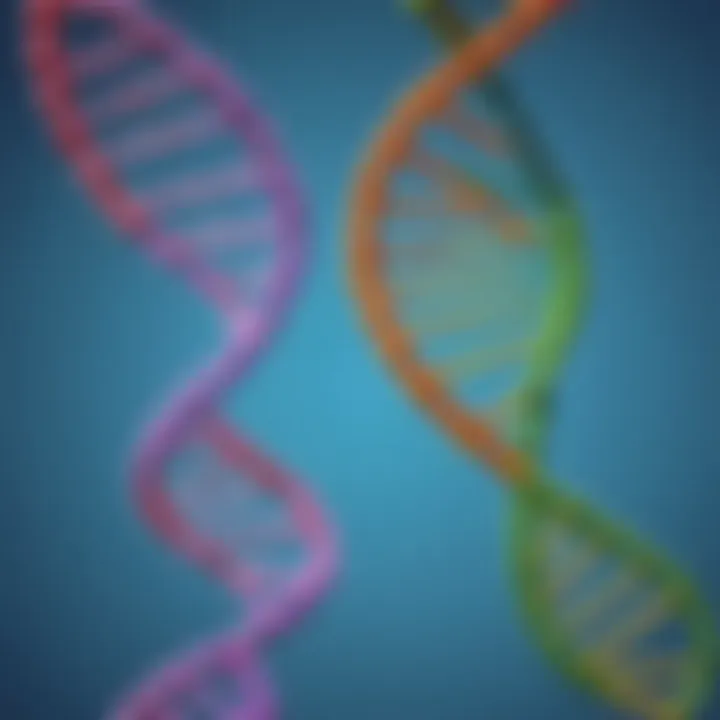
Intro
Genetic engineering is a fascinating field that holds the key to understanding how life works. It involves modifying an organism's DNA to achieve desired traits. This topic plays an important role in many areas, from medicine to agriculture. As we explore this subject, we will discover its fundamental concepts, technological advancements, applications, ethical considerations, and future implications. The aim is to present this complex topic in an accessible way for children, educators, and parents alike.
Science Fun Facts
Genetic engineering is not just a modern phenomenon. Here are some interesting facts to get you started:
- The first successful genetic engineering experiment occurred in 1973 when scientists modified the DNA of a bacterium to produce human insulin.
- Modern crops like corn, soybeans, and cotton are often genetically engineered to resist pests and herbicides, making farming more efficient.
- Did you know? Genetic engineering can help us produce medicines more quickly. For example, scientists have genetically modified bacteria to produce vaccines.
"Genetic engineering has the potential to change the world for the better, from improved food to life-saving medicines."
These facts provide just a glimpse into the powerful effects of genetic modification.
Interesting Trivia and Facts
- Human DNA is 99.9% similar among individuals. This small difference accounts for all the uniqueness seen in people.
- Scientists can edit genes using special tools called CRISPR. This method is fast and precise, making it a popular choice for researchers.
Quirky Science Stories
One of the most exciting stories in genetic engineering involves a glowing fish known as the GloFish. These fish were originally created to help detect water pollution. Their unique gene modification causes them to glow in different colors under black light. This showcases how genetic modification can lead to interesting applications in science and ecology.
Amazing Science Records
- The largest genetically modified organism is the Arachis hypogaea, or the peanut. Some varieties are engineered to be more resistant to pests and diseases.
- The world's first genetically modified human embryos were reported by scientists in 2017, marking a critical milestone in the field.
Thought-Provoking Questions
- What are the potential benefits and risks of genetically modifying crops?
- How might genetic engineering change the future of medicine?
These questions prompt critical thinking and can encourage interesting discussions about the implications of genetic engineering in our society.
Intro to Genetic Engineering
Genetic engineering is a significant area of study with vast implications for many fields. It involves modifying the genetic material of organisms to achieve desired traits or outcomes. This introduction serves as a foundation for understanding why genetic engineering is essential today.
By grasping the core principles and techniques involved in genetic engineering, readers can appreciate its impact on medicine, agriculture, and the environment. The benefits of genetic engineering are numerous, including improved crops, new treatments for diseases, and solutions to environmental problems. However, there are also concerns regarding its ethical implications and long-term effects on biodiversity.
Definition and Scope
Genetic engineering can be defined as the manipulation of an organism's DNA to alter its characteristics. Scientists use various techniques to add, delete, or modify genes. The scope of genetic engineering extends across many disciplines, such as agriculture, medicine, and ecological research. For example, genetically modified organisms (GMOs) are common in agriculture, where scientists create plants that are resistant to pests or have enhanced nutritional values. In medicine, genetic engineering plays a crucial role in developing gene therapy techniques that aim to treat genetic disorders by directly modifying faulty genes.
Historical Background
The history of genetic engineering dates back to the early 20th century, even before the term was popularized. The discovery of DNA's structure in 1953 by James Watson and Francis Crick paved the way for the field. In the early days, traditional methods of selective breeding were used to enhance traits in plants and animals. However, in the 1970s, scientists developed new techniques such as recombinant DNA technology, which allowed for more precise modifications. Since then, advancements like CRISPR-Cas9 technology have revolutionized the field, enabling researchers to edit genes with increased accuracy and efficiency. Understanding this historical context is crucial, as it showcases the evolution of scientific thought and technology that has shaped modern genetic engineering.
The Science of Genetics
DNA and Genes
Structure of DNA
The structure of DNA is a double helix, composed of two long strands wrapped around each other. Each strand is made up of smaller units called nucleotides. The main components of a nucleotide are a phosphate group, a sugar molecule, and a nitrogenous base. There are four types of nitrogenous bases in DNA: adenine, thymine, cytosine, and guanine.
Key characteristics of DNA's structure include its stability and the way it stores genetic information. This makes DNA a popular choice for studying genetics. Its unique feature is how it can replicate itself accurately, ensuring that genetic information is preserved through generations.
Advantages of the double helix structure in DNA allow for effective error checking during replication, minimizing mutations. However, this stability also means it can be hard to change genetic material quickly, which can be a disadvantage when rapid adaptations are needed.
Function of Genes
Genes, the segments of DNA, serve as the instructions for building proteins. They determine many traits in an organism, influencing characteristics such as eye color or disease resistance. Each gene has a specific location on a chromosome and can vary in its sequence among different individuals, leading to variations in traits.

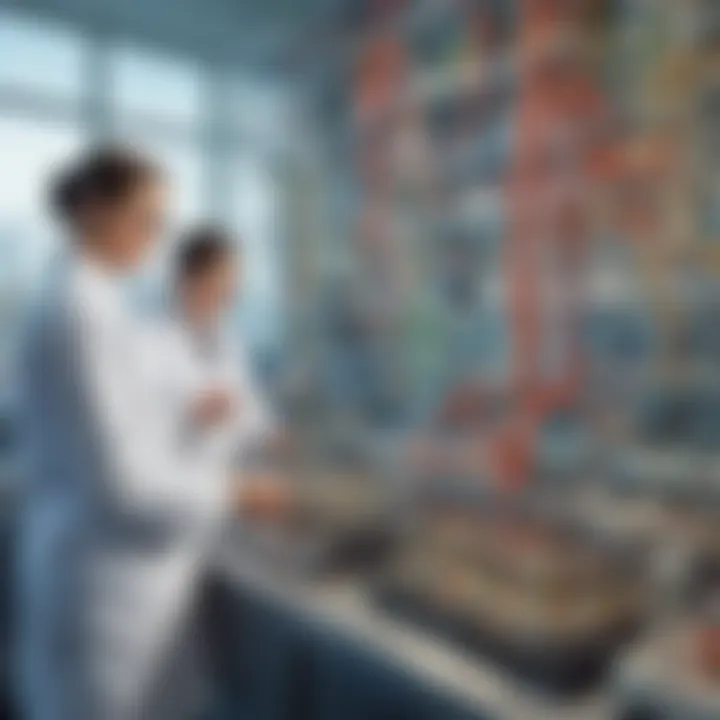
The key characteristic of genes is their role as templates for protein synthesis, making them vital in determining organism function. This ability to instruct cellular machinery makes genes a beneficial focal point in the article.
A unique feature of genes is that slight changes in their sequences, known as mutations, can lead to significant changes in traits or functions. The advantage of studying gene function lies in the potential to identify target genes for genetic engineering applications. However, unintended mutations can also pose risks, complicating genetic modifications.
Genetic Variation
Genetic variation is the diversity in gene frequencies among individuals in a population. This variation is essential for evolution and plays a critical role in how species adapt to changing environments. Different forms of a gene, called alleles, contribute to this variation.
Genetic variation provides several benefits. It allows for the survival of species in different ecosystems. It also facilitates advancements in agriculture, medicine, and other fields. Genetic engineering aims to harness beneficial variations while minimizing adverse effects.
By understanding genetic variation, scientists can develop targeted approaches in genetic engineering, leading to innovations that improve health outcomes and agricultural efficiency.
"Genetic variation is essential for the survival and adaptability of species. Without it, there would be less diversity and resilience in ecosystems."
Techniques in Genetic Engineering
Techniques in genetic engineering form the backbone of advancements in this field. They provide the methods and tools necessary for manipulating genes. Understanding these techniques is essential as they play a critical role in both research and practical applications. Moreover, each technique offers different benefits and presents unique considerations. This knowledge sets the stage for evaluating the contributions of genetic engineering to science, agriculture, and medicine.
CRISPR-Cas9
Mechanism of Action
The CRISPR-Cas9 system is a revolutionary tool in genetic engineering. This technique allows scientists to edit specific parts of the genome. The key characteristic of CRISPR-Cas9 is its precision. It uses a guide RNA to locate a target DNA sequence. Once found, the system can cut the DNA at that specific location. This ability to make targeted changes to the genome is its biggest strength.
A unique feature of CRISPR-Cas9 is its relatively low cost and ease of use. Compared to older methods, this technique allows for quicker editing and analysis. However, there are also concerns. Off-target effects may occur, leading to unintended consequences in the genome, which may change how certain genes function in ways that are not fully understood.
Applications in Research
The applications of CRISPR-Cas9 in research are vast and transformative. It has changed how scientists approach genetic studies. The key characteristic is its versatility across different organisms. This allows researchers to study gene functions in various contexts.
A unique feature of using CRISPR-Cas9 in research is its capability to assemble comprehensive genetic models. It helps in understanding diseases better and can lead to breakthroughs in treatment. Its advantages include reduced time and resources needed for gene editing. Yet there are pitfalls to consider, such as ethical questions surrounding its use and potential long-term effects of gene edits.
Gene Cloning
Gene cloning is another fundamental technique in genetic engineering. It enables the replication of specific genes, facilitating detailed study. This process involves isolating a gene and inserting it into a vector. The vector then replicates, producing multiple copies of the gene. One main benefit of gene cloning is its ability to produce large quantities of specific genes. This aids in research and application in various fields, including medicine.
However, gene cloning's efficiency does not come without concerns. There are ethical issues surrounding the use of cloned genes, particularly regarding genetic diversity and potential impacts on ecosystems. The conversation around gene cloning is complex and requires careful consideration.
Transgenic Organisms
Definition and Examples
A unique aspect of transgenic organisms is the introduction of foreign DNA. This allows scientists to study gene function and its effects on the organism, leading to notable advancements in biotechnology. Yet, there are disadvantages, such as public skepticism regarding safety and ethics of transgenic organisms which need careful navigation.
Role in Agriculture
Transgenic organisms play a significant role in agriculture. Their contribution to enhancing crop traits is increasingly recognized. For example, genetically modified crops can be designed to withstand harsh conditions like drought and pests. This helps in meeting global food demands and ensures food security.
The key characteristic of transgenic organisms in agriculture is their potential to increase yield and resilience. Through genetic modification, farmers can grow crops more efficiently. The advantages are apparent; however, it requires regulatory scrutiny and societal acceptance. The implications of using transgenic organisms must be discussed thoroughly to address public concerns and ensure sustainable practices.
Applications of Genetic Engineering
The field of genetic engineering plays a crucial role in various sectors, including medicine, agriculture, and environmental science. Understanding these applications helps illustrate the potential benefits and challenges presented by genetic modification. In this section, we will explore how genetic engineering supports innovation and addresses key issues in each domain.
Medical Advances
Gene Therapy
Gene therapy represents a significant advancement in medicine. It focuses on correcting genetic defects in individuals by introducing, removing, or altering genetic material within their cells. This technique can potentially treat diseases caused by mutations in specific genes.
A key characteristic of gene therapy is its specificity. It directly targets the root cause of genetic disorders, rather than merely managing symptoms. This focus makes it a promising choice for treating conditions like cystic fibrosis and certain types of cancer.
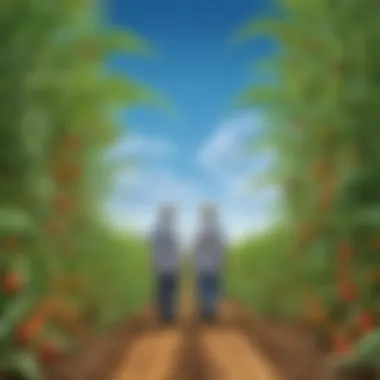
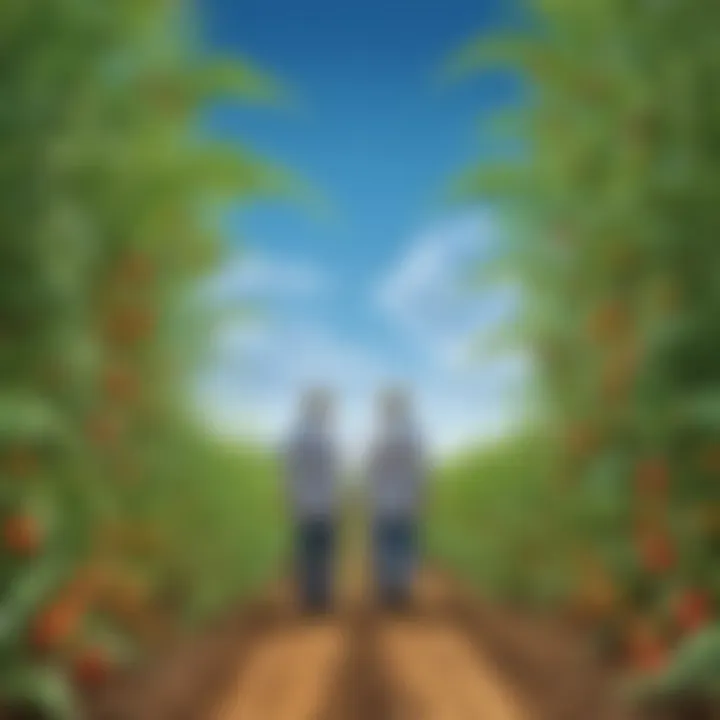
However, gene therapy has unique features that present both advantages and disadvantages. One advantage is its potential to provide long-lasting solutions for genetic disorders. Conversely, some challenges include the technical complexity involved in delivering therapies and the risk of unintended effects on other genes.
Production of Insulin
The production of insulin through genetic engineering has transformed diabetes treatment. By inserting the human insulin gene into bacteria, scientists can produce insulin more efficiently than ever before. This recombinant DNA technology allows for large-scale production of insulin, crucial for managing diabetes.
The key characteristic of this process is its efficiency. Unlike extracting insulin from animal sources, which was common in the past, this method ensures a consistent and readily available supply. This innovation is particularly beneficial for patients who rely on insulin to manage their condition.
Despite its advantages, there are considerations to keep in mind. While genetically engineered insulin is effective, there have been discussions related to the production process and the need for ongoing regulation to ensure safety and quality.
Agricultural Innovations
Crops with Enhanced Traits
Crops with enhanced traits are a direct result of genetic engineering's application in agriculture. These crops are developed to withstand pests, diseases, and environmental stresses, which can lead to higher yields. For instance, Bt cotton and Bt corn have been engineered to produce a protein that is toxic to specific pests, reducing the need for chemical pesticides.
A key feature of these crops is their resilience. They can thrive under conditions that would typically hinder traditional varieties. This characteristic makes them a favorable choice for addressing global food security.
Unique features of such crops include improved nutritional profiles and reduced environmental impact. However, there are concerns regarding the long-term ecological effects and potential resistance that pests might develop over time.
Impact on Food Production
The impact on food production is profound due to genetic engineering. Introducing genetically modified organisms (GMOs) can increase productivity and sustainability. These innovations often address food scarcity issues in various regions of the world.
The main characteristic of this impact is the potential for higher efficiency. Farmers can grow more food on less land, which is essential as the global population continues to grow. This trait makes it a popular focus in discussions about agricultural practices.
Nevertheless, the unique challenges include public perception and regulatory hurdles. Some consumers are hesitant about GMOs, leading to calls for transparency and labeling in food production.
Environmental Applications
GMO Microorganisms
GMO microorganisms are engineered for various environmental applications. They can be used in bioremediation, which involves using microbes to clean up pollutants in soil and water. For example, certain bacteria can be modified to degrade oil spills more effectively.
The distinguishing feature of GMO microorganisms is their adaptability. They can be tailored to specific pollutants, improving efficiency in environmental clean-up efforts. This property makes them a popular solution in addressing environmental challenges.
However, there are concerns regarding ecological impact. The introduction of modified organisms into natural ecosystems can have unforeseen consequences, making regulation and assessment necessary.
Biosolutions to Pollution
Biosolutions to pollution use living organisms to address environmental issues. This approach can include using plants and microorganisms to absorb heavy metals from contaminated sites. It is an innovative way of mitigating pollution without harsh chemicals.
The key characteristic of this solution is sustainability. By utilizing natural processes, biosolutions can offer an eco-friendly alternative to traditional remediation methods. This makes it a favorable choice for addressing pollution in a gentler manner.
Despite its benefits, there are some drawbacks. The slow nature of biological processes can make biosolutions less effective in situations requiring immediate results. Long-term commitments and monitoring may also be necessary to ensure effectiveness over time.
Ethical Considerations
The field of genetic engineering raises substantial ethical questions that compel society to reflect on the implications of its advancements. Understanding these considerations is essential for anyone engaging with the topic, as they shape public opinion and policy. This section will explore debates surrounding genetic modification and the consequences it may hold for both humanity and the environment.
Debates on Genetic Modification
Discussions on genetic modification can be polarizing. On one side, advocates highlight the potential benefits such as improved health outcomes and agricultural efficiency. This includes the possibility of eliminating genetic diseases and enhancing food security. Many argue that modifying organisms to be more resilient ensures we can meet growing global needs.
Conversely, opponents voice concerns about the unforeseen consequences. They often address the ethics of altering the genetic makeup of living organisms. Questions arise such as: What rights do organisms have? Can humans ethically play the role of "designer"? Such discussions are vital as they unearth deeper societal beliefs about nature and the limits of human intervention.
Consequences of Genetic Engineering
As genetic engineering progresses, understanding its potential consequences for individuals and ecosystems becomes crucial. With any new technology comes risks and ethical dilemmas.
Human Rights Concerns
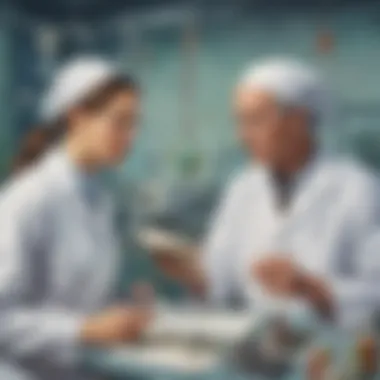
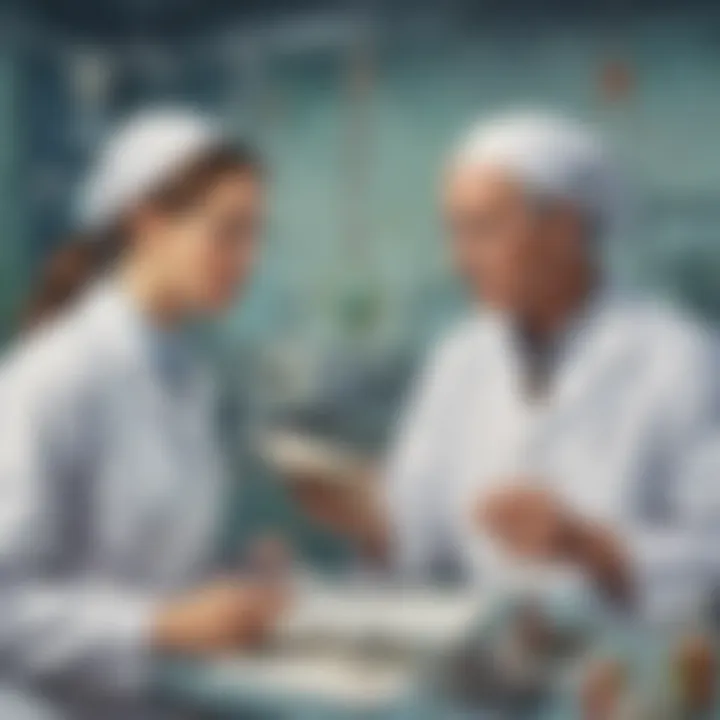
Human rights concerns in genetic engineering primarily focus on issues of access and equity. As advanced therapies emerge, questions arise about who benefits from such innovations. If treatments are expensive, will only the wealthy have access? This disparity raises significant ethical issues about justice and fairness.
A key characteristic of these concerns is their relation to health equity. Ensuring that everyone, regardless of their social or economic status, has access to genetic advancements is vital. If inequality persists, it creates a divide that can lead to social unrest and a sense of injustice. Understanding human rights in this context is beneficial for fostering discussions on equitable access.
Moreover, it confronts the unique feature of consent. Individuals must have the right to choose if they want to engage with genetic engineering. Informed consent ensures people understand the risks and benefits involved. However, it can be complicated, especially for children and vulnerable populations. Therefore, careful consideration is required to protect rights.
Impacts on Biodiversity
The impacts of genetic engineering on biodiversity present another pressing ethical dilemma. Genetic modifications can lead to enhanced species, but they also run the risk of disrupting existing ecosystems. For example, the introduction of genetically modified organisms into an environment might outcompete native species, leading to extinction.
A defining characteristic of these impacts is the balance between innovation and preservation. While advancements can lead to more robust crops that resist pests, they can also reduce genetic variation in natural populations. Maintaining biodiversity is essential for ecosystem stability and resilience. This discussion is important as it links back to ethical considerations regarding stewardship of our environment.
The unique feature of biodiversity impacts is their complex nature. Effects may not be immediately visible and can take generations to unfold. Thus, caution is crucial when implementing genetic technologies. Understanding these implications can help frame policies that prioritize both innovation and ecological health.
"The true cost of genetic engineering is not just a matter of technology, but one of ethics and long-term environmental impact."
In summary, the ethical considerations surrounding genetic engineering are vital components to their successful integration into society. By engaging with these complexities, we can better navigate the future of genetic advancements.
Future Perspectives
Understanding the future perspectives in genetic engineering is essential for grasping how the field will evolve. Genetic engineering promises numerous opportunities and challenges that could reshape various industries, from medicine to agriculture. As technology advances, it is important to consider potential developments and societal implications that arise from these changes.
Potential Developments
The horizon of genetic engineering is filled with potential advancements. Scientists and researchers are continuously working on refining existing technologies and developing new methodologies. Here are some key areas of focus:
- Gene Editing Tools: Innovations like CRISPR-Cas9 are likely to be enhanced further. New techniques could offer greater precision, reduce off-target effects, and expand the range of editable sequences.
- Synthetic Biology: This field involves designing new biological parts and systems. Future developments may lead to custom organisms that can produce biofuels, pharmaceuticals, and other valuable products more efficiently.
- Personalized Medicine: Genetic engineering could revolutionize healthcare by allowing treatments tailored to an individual’s genetic makeup. This approach might side-step traditional methods that are not as effective for everyone.
- Agricultural Improvements: Advancements in creating crops that are resistant to diseases or have improved nutritional profiles could significantly impact food security and sustainability.
Societal Implications of Advances
As genetic engineering continues to progress, it bears significant societal implications that require careful consideration.
Education and Awareness
Education and awareness are pivotal in shaping public understanding of genetic engineering. An informed society is better equipped to engage in discussions about its impact.
- Key Characteristic: Initiatives aimed at increasing understanding can be tailored to different age groups, making complex topics accessible for younger audiences.
- Benefit: Such programs help demystify genetic engineering, encouraging critical thinking and enabling citizens to make informed decisions.
- Unique Feature: Programs that include hands-on learning experiences can foster curiosity and inspire the next generation of scientists and engineers.
Regulatory Frameworks
Effective regulatory frameworks are crucial in managing the ethical and safety aspects of genetic engineering.
- Key Characteristic: Regulations ensure that developments in genetic technologies are both safe and ethical.
- Benefit: A robust framework can enhance public trust in biotechnologies, paving the way for acceptance and support.
- Unique Feature: By prioritizing transparency and accountability, regulations can help address public concerns over genetic manipulation and its consequences.
In summary, the future of genetic engineering holds transformative potential. Its developments promise to revolutionize numerous fields, but this also necessitates careful consideration of societal impacts and adequate regulation to ensure that advancements are responsible and ethical. Informed discussions and education on genetic engineering will be key in navigating its future.
Culmination
In the realm of genetic engineering, it becomes vital to highlight the benefits gained through scientific advancement. These include the potential for medical breakthroughs, agricultural improvements, and environmental solutions. Each subsection dissected in the article plays a role in painting a comprehensive picture of the discipline's scope, including its promises and ethical challenges.
The importance of ongoing discussion around genetic engineering cannot be overstated. As society navigates the fast-changing landscape of biotechnology, an informed understanding fosters critical engagement among young learners and their guardians.
Additionally, recognizing the ethical dimensions discussed, such as the implications of genetic modifications, aids in preparing future citizens to make informed decisions. Ethical discussions are a cornerstone in evaluating genetic advancements' societal impacts. This holistic view nurtures not just awareness but a deeper responsibility towards the world.
Recap of Key Concepts
As we reach the conclusion of our exploration, it is pivotal to recap the main points discussed throughout the article. Here are the highlights:
- Definition of Genetic Engineering: A process involving the manipulation of an organism's DNA to achieve desired traits.
- Techniques: Innovations like CRISPR-Cas9, gene cloning, and the creation of transgenic organisms.
- Applications: Significant impacts in medicine, agriculture, and environmental approaches.
- Ethical Considerations: The moral debates surrounding genetic modification and its effects on biodiversity and human rights.
- Future Perspectives: Anticipated advancements and their societal implications, including the need for proper educational frameworks and regulations.
These concepts provide a foundation for understanding the intricate world of genetics. Each topic interlinks with the others, forming a vast network of implications that extend beyond science into ethical realms.
Encouragement for Further Exploration
Encouraging further exploration in genetic engineering is crucial for young minds. As knowledge evolves, so does the potential for groundbreaking applications. Here are ways to continue the learning journey:
- Engage with Resources: Websites like Wikipedia or Britannica provide extensive information on genetics and biotechnology.
- Participate in Workshops: Many local science centers offer workshops and educational programs about genetic science suitable for children.
- Read Books: There are numerous age-appropriate books available that discuss genetics in accessible language.
- Discuss with Experts: If possible, attend talks by scientists and educators in the field to gain firsthand perspectives.
- Explore Online Forums: Websites like Reddit can connect to discussions on genetic engineering where questions can be asked and answered.
Through continuous learning and curiosity, the next generation will be equipped to approach the challenges and opportunities presented by genetic engineering thoughtfully. This proactive stance will empower them to become informed citizens who can contribute positively to society's scientific discourse.