Unraveling the Physics of Roller Coaster Science Projects
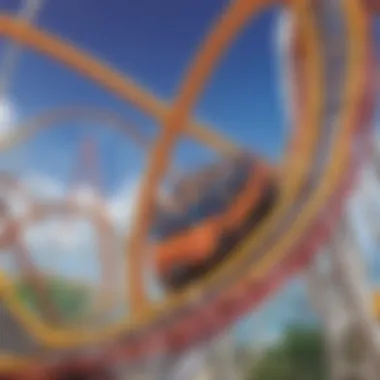
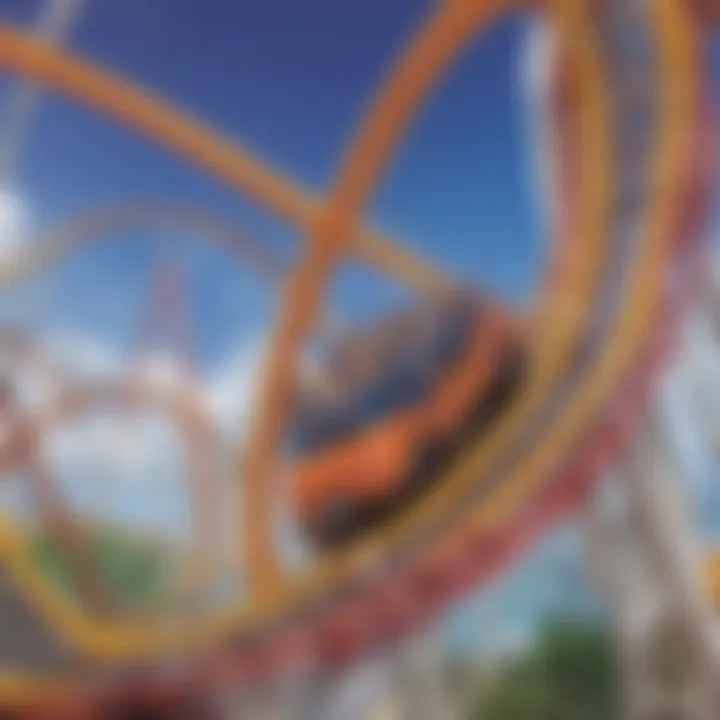
Intro
Roller coasters have a unique attraction. They not only offer thrilling rides but also serve as a practical platform to explore various scientific principles. As we analyze their design, we encounter concepts of physics, engineering, and mathematics that unwind the secrets of motion. Understanding these fundamentals can lead to captivating projects for young science enthusiasts.
This guide presents an avenue to delve into roller coaster science with detailed instructions and explanations. Parents and educators can utilize this resource to create enriching experiences that spark curiosity in children. Each segment aims to illuminate the principles behind roller coasters while fostering hands-on exploration.
Preamble to Roller Coaster Science
Understanding the mechanics behind roller coasters provides insight into the fascinating world of physics and engineering. This section lays out the groundwork for learning about these thrilling rides. Roller coasters are not just amusement park attractions; they serve as real-life applications of various scientific principles.
An exploration into roller coaster science encompasses concepts such as energy conservation, gravity, and motion, which are pivotal in the design and function of these rides. By deconstructing how roller coasters work, learners can appreciate the combination of creativity and physics required to construct these models. This exploration invites critical thinking and inquiry, which are essential skills in science education.
By engaging with roller coaster projects, students better grasp elements of forces, energy, and structural integrity. Furthermore, constructing a model allows students to witness firsthand the application of theories from textbooks in a tangible way. The principles learned through this medium can be vital for anyone considering a future in science or engineering fields.
"Using hands-on projects like roller coasters facilitates a deeper comprehension of complex scientific concepts."
Thus, roller coaster science projects not only ignite curiosity but also enhance problem-solving skills among young learners. The interdisciplinary nature of this topic connects various branches of science, making it an ideal subject for educational exploration.
Understanding the Physics of Roller Coasters
Roller coasters elegantly demonstrate fundamental principles of physics. The physics involved is not just theoretical; it is present and observable in every twist and turn of a roller coaster. To understand how they function, one must delve into two main types of energy: potential energy and kinetic energy.
When a roller coaster climbs to the peak of a hill, it accumulates potential energy. This potential energy is influenced by the height of the coaster and the weight of the riders. As the coaster begins its descent, this potential energy transforms into kinetic energy, which is the energy of motion. The faster the coaster moves, the more kinetic energy it has.
The interplay between these two types of energy forms the basis of roller coaster design. A well-designed ride carefully balances height and speed to create thrills while maintaining safety.
Key Scientific Principles at Play
Several scientific principles come into play when considering roller coasters. These include:
- Inertia: This is the tendency of objects to remain in motion or to remain at rest. Riders feel this force as they go through loops and turns.
- Gravity: It pulls the coaster down, enabling the conversion of potential energy into kinetic energy.
- Friction: This force acts against the motion of the coaster. Designers must account for friction when calculating the coaster's speed and safety.
Understanding these principles can clarify why roller coasters operate as they do. Young learners will find it easier to appreciate how forces impact their ride experience, fostering a genuine interest in how science shapes the world around them.
Designing Your Roller Coaster Model
Designing a roller coaster model is a vital part of exploring the science behind roller coasters. It allows students and enthusiasts to apply physics concepts in a more hands-on way, fostering both creativity and problem-solving abilities. The model serves not only as a demonstration of theoretical principles but also as a foundation for practical application. Understanding how to design a functioning roller coaster helps learners grasp the significance of materials, energy transfer, and the role of gravity. This part of the project is crucial for creating a successful model that reflects real-world physics and engineering concepts.
Selecting Appropriate Materials
Choosing the right materials for building a roller coaster model can significantly impact its performance and durability. Different materials come with unique characteristics that make them suitable for specific aspects of design. This section will explore three popular options: paper and cardboard, wood and straws, and plastic and metal components.
Paper and Cardboard
Paper and cardboard are light and easy to work with. They are often chosen for their accessibility and cost-effectiveness. These materials allow for flexibility in design, making it simple to cut, bend, and shape the components of the roller coaster. A key characteristic is that they can produce a decent structural design with minimal weight. Using paper and cardboard is a beneficial choice for beginners, especially elementary school children. One unique feature of paper is that it is readily available and recyclable.
However, they do have some limitations. For instance, paper and cardboard can struggle to support heavier elements of the design, potentially leading to structure instability over time. They may also degrade when exposed to moisture, which can limit their longevity outdoors.
Wood and Straws
Wood and straws present a more versatile approach while still remaining relatively lightweight. The key characteristic of wood is its increased rigidity, which allows for more complex designs and greater height. Straws, on the other hand, can be utilized for smooth track creation. This combination allows for diverse roller coaster effects. The torque and tension in wooden structures often offer realism in models.
However, wood requires more tools and skills to manipulate compared to paper. The unique feature of using straws is their ability to create smooth curves in the track, but they must be carefully aligned to prevent misalignment during testing. Additionally, wood may increase costs and complexity in construction for beginners.
Plastic and Metal Components
Plastic and metal components are more advanced choices for roller coaster modeling. They offer durable and long-lasting designs, reducing the risk of wear and tear during tests. A key characteristic of plastic is its ability to mold into vibrant colors and intricate shapes, enhancing visual appeal. Metal, on the other hand, provides exceptional strength, which is essential for high-speed tracks.
These materials are a beneficial selection for more experienced builders aiming for realism in their projects. A unique feature of plastics is that they can connect easily with different joints or fasteners, which is valuable during construction.
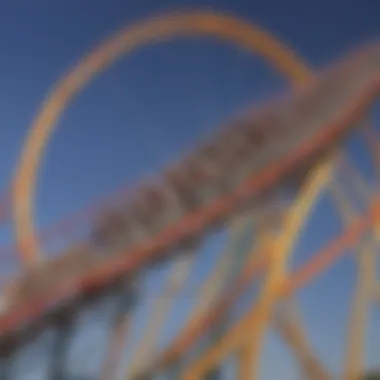
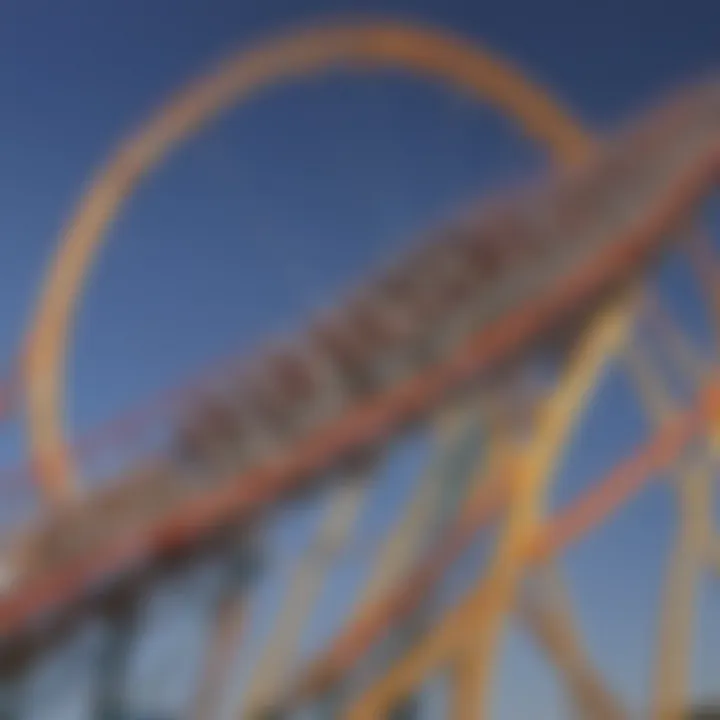
However, there are downsides. These materials can be more costly than wood or paper. Also, working with metal requires safety precautions and advanced tools, which may not be suitable for all age groups.
Creating a Blueprint
Creating a blueprint is the next essential step after selecting materials. The blueprint serves as a guide for building the model, detailing each section of the track, the height of hills, and the angles of turns. This planning phase is critical as it allows makers to visualize the project and ensure the model is feasible. A thoughtful blueprint helps in anticipating challenges and making necessary adjustments before physically constructing the roller coaster.
Understanding Energy Transfer
Energy transfer is a core concept in the science of roller coasters. It helps explain how these thrilling rides operate, focusing on how energy changes forms as the coaster travels along its track. Understanding energy transfer not only lays the groundwork for building a successful roller coaster model but also provides insight into broader principles of physics that apply in numerous life situations.
One significant benefit of grasping energy transfer is appreciating how potential energy and kinetic energy work together. For students and educators, recognizing these concepts can inspire curiosity about the laws of nature that govern motion and energy. This knowledge is essential for designing models, running experiments, and appreciating the sophisticated engineering behind real-life roller coasters.
Potential Energy Explained
Potential energy is the energy that an object has because of its position or condition. In the context of roller coasters, potential energy is primarily influenced by height. For instance, when a coaster rises to the peak of a hill, it stores significant potential energy.
The amount of potential energy can be calculated using the formula:
Potential Energy (PE) = mass (m) × gravitational acceleration (g) × height (h)
This equation highlights how the height and mass of the coaster impact the energy stored. As the coaster ascends, it gains potential energy, and vice versa when it descends.
- Key points to remember about potential energy:
- Higher elevations correspond to higher potential energy.
- Mass plays a critical role; heavier coasters store more energy.
- It converts to kinetic energy as the coaster drops.
Kinetic Energy Dynamics
Kinetic energy refers to the energy an object has due to its motion. In roller coasters, as potential energy converts to kinetic energy during a drop, the coaster accelerates. This transformation is essential for creating thrilling experiences.
Kinetic energy can be described with the formula:
Kinetic Energy (KE) = 0. × mass (m) × velocity²
As the coaster speeds up, the kinetic energy increases while the potential energy decreases.
- Important aspects of kinetic energy:
- Speed directly affects the amount of kinetic energy.
- Safety concerns arise from high speeds, as they affect the forces experienced by riders.
- Understanding these dynamics is crucial for designing tracks that provide excitement while ensuring safety.
Conversion of Energy in Roller Coasters
The conversion of energy between potential and kinetic forms is fundamental to roller coaster operation. As a ride begins, the coaster is lifted to a high point, accumulating potential energy. When it rolls down, this stored energy converts to kinetic energy, allowing the coaster to reach high speeds.
This cycle continues throughout the ride. At various points, energy transforms back and forth between these two forms, maintaining the momentum necessary for the coaster's operation.
Understanding how energy converts lays the groundwork for various engineering and design considerations.
- Key insights on energy conversion:
- Efficiency of energy transfer affects ride duration and speed.
- Friction and air resistance can lead to energy loss, requiring careful design choices.
- Innovative coaster designs often focus on optimizing energy conversion for the best performance.
The Role of Gravity in Roller Coaster Functionality
Understanding the role of gravity is essential when exploring roller coaster functionality. Gravity is a natural force that pulls objects toward each other. On Earth, it pulls everything towards the center of the planet. In roller coasters, gravity acts as a primary force influencing not just speed, but also the design and safety of the ride.
One crucial aspect is how gravity affects the motion of the roller coaster cars. When the cars ascend a hill, they gain potential energy due to their height. As they descend, this potential energy transforms into kinetic energy, which accelerates the cars. This transition of energy governed by gravitational force creates thrilling experiences for riders.
How Gravity Affects Motion
Gravity significantly influences the speed and movement patterns of roller coaster cars. When a car climbs to a higher elevation, it stores energy, which is known as potential energy. The higher the car goes, the more potential energy it accumulates. At the peak of the hill, when the cars begin to descend, gravity pulls them down, converting that stored energy into kinetic energy. This is why the cars move faster in the descent compared to the ascent.
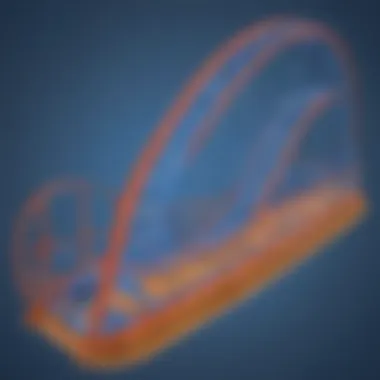
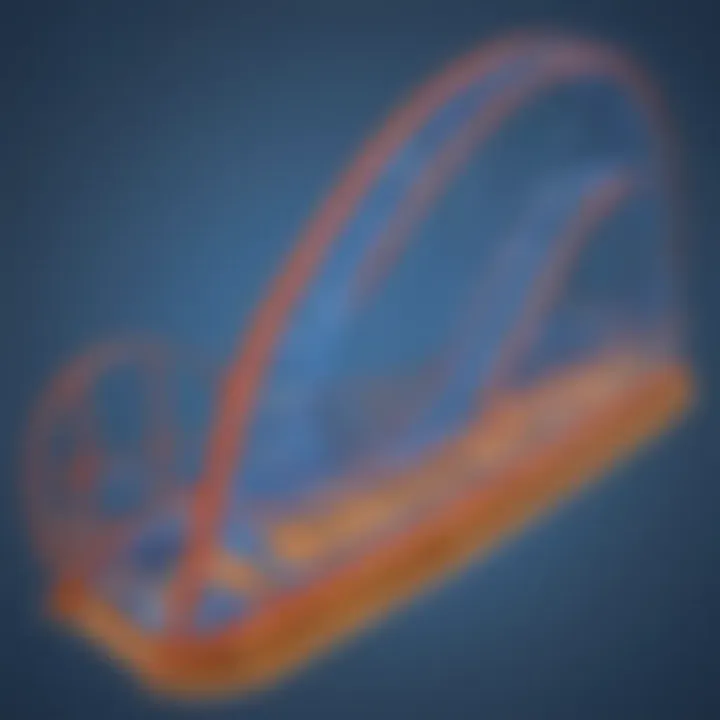
- Potential Energy: Stored energy at height
- Kinetic Energy: Energy of movement on descent
How fast a roller coaster moves is determined by the height it starts from and how steep the drop is. The steeper the drop, the more gravitational force acts on the car, resulting in a faster speed. However, gravity does not just make the cars go faster; it also creates challenges. Designers must account for gravity's effects to ensure passenger safety throughout the ride.
Incorporating Gravity in Model Design
When building a roller coaster model, understanding gravitational forces is crucial. Designers should consider how elevations and drops affect the movement of their model. Here are some important factors to keep in mind:
- Track Height: Higher tracks lead to greater potential energy.
- Angle of Descent: Steeper angles create faster speeds due to stronger gravitational effects.
- Material Weight: Heavier materials may influence how gravity affects your model.
To implement gravity effectively in your roller coaster model, you can:
- Sketch a blueprint that includes varying heights.
- Use materials that provide stability while allowing smooth motion of the cars.
- Test different heights and angles by adjusting sections of the track after initial construction to observe changes in speed and safety.
By incorporating these elements, you can better understand how gravity functions in your model and creates a realistic simulation of actual roller coasters. The effects of gravity are crucial in ensuring that the ride is both exciting and safe for potential riders.
"The interplay of gravity and design is what makes roller coasters both thrilling and scientific."
As we can see, gravity is not just a force; it is a vital component that intertwines with engineering principles, making it an essential topic to discuss in roller coaster science projects.
Creating the Roller Coaster Simulation
Creating a simulation of a roller coaster is not just a fun activity, it is an essential part of understanding the principles of physics and engineering. This section covers two critical areas: building the track layout and testing different designs. In creating a roller coaster, students grasp the interplay between design, physics, and safety. They learn to visualize concepts that are often abstract.
Building the Track Layout
The track layout is the foundation of your roller coaster simulation. It defines how the ride will operate, including twists, turns, and drops. When designing your track, consider the following:
- Curvature: Use gradual curves to ensure your cars maintain speed without losing momentum.
- Elevation: Elevated sections can increase potential energy, leading to thrilling drops that convert energy into speed.
- Support Structure: A stable structure is crucial for safety and function. It needs to hold the weight of the track and the vehicles securely.
Select a suitable material for the track. Cardboard can serve well for initial designs due to its light weight and ease of manipulation. However, for more durability, consider using wooden dowels or plastic tubing.
"The beauty of building a roller coaster simulation lies in the execution of creativity rooted in scientific principles."
Sketching your layout on paper can help visualize the final product. Create multiple designs and discuss how each will affect the ride's speed and experience. Keep in mind that the most complex designs may not always be the best.
Testing Different Designs
Once you build your initial track, testing is imperative. This phase allows you to see what works and what does not, providing a hands-on learning experience. Different designs will showcase various aspects of kinetic and potential energy. During testing, consider these points:
- Speed: Measure how fast the cars travel down various slopes. Notice how steepness affects speed and momentum.
- Stability: Ensure your structure can handle the forces acting on it. If your ride goes too fast through a loop, it may derail.
- Rider Experience: Ask participants to describe their experience on different test runs. Is there enough thrill? Are there sections that feel too slow?
Adjust designs based on your findings. You can run multiple trials, altering one variable at a time. This allows for a scientific approach to problem-solving. Each iteration of your test brings you closer to a refined simulation.
Mathematics in Roller Coaster Projects
Mathematics plays a crucial role in the design and construction of roller coaster projects. It provides the necessary tools to analyze motion, calculate speeds, and measure trajectories. Understanding these mathematical principles allows students to grasp the underlying science behind rides that bring thrill and excitement.
Through hands-on projects, young learners can appreciate how mathematics is not just abstract theory but a practical tool. Skills gained through these activities can be foundational for more advanced studies in physics and engineering. Furthermore, engaging with these concepts promotes analytical thinking and problem-solving abilities.
Calculating Speed and Velocity
Calculating speed and velocity is an essential part of roller coaster projects. Speed is the distance traveled over time. In contrast, velocity takes the direction of movement into account, giving a clearer picture of how a roller coaster operates. To measure speed and velocity, students can use simple formulas.
For example, speed can be calculated using:
Using this formula allows students to determine how quickly a roller coaster car travels down the track.
Steps for calculating speed would include:
- Measuring the length of the track to determine the distance.
- Timing how long it takes to travel that distance.
- Dividing the distance by the time.
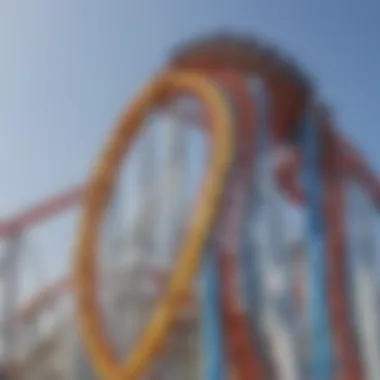
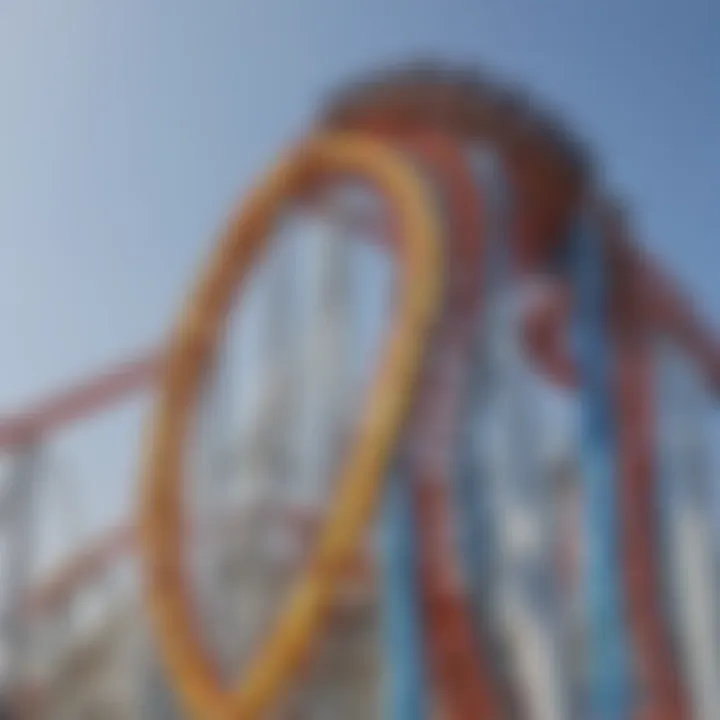
This hands-on approach teaches them how speed affects the experience of a ride. A roller coaster that goes too fast may be thrilling but could also be unsafe. Therefore, understanding how to calculate these figures is important in engineering a safe ride.
"Math is not just numbers; it’s the framework of our world and how we understand motion in it."
Measuring Height and Distances
Measuring height and distances is equally vital. The height of a coaster affects the potential energy, energy that can be converted to kinetic energy as the ride descends. Understanding how to accurately measure these parameters can enhance project design.
To measure the height of a roller coaster model, students can use a ruler or measuring tape. It can also be good practice to mark down the height at different points on the track.
Steps for measuring height and distances may include:
- Using a device, like a level or protractor, to ensure vertical accuracy.
- Recording measurements at multiple points along the track.
- Creating a data chart to illustrate the changes in height and how they affect speed.
Incorporating these calculations into roller coaster projects emphasizes the connection between mathematics and practical applications. Students realize how essential these measurements are in creating a functional and safe roller coaster model.
Safety Considerations in Roller Coaster Engineering
The safety of roller coasters is a paramount aspect of their design and operation. In any engineering project, especially one involving significant speeds and heights, understanding how safety measures apply can create a foundation for subsequent explorations. Safety considerations in roller coaster engineering encompass various elements that ensure not only rider enjoyment but also their protection against potential hazards.
When constructing roller coaster models, it is essential to think about the structural integrity and how forces affect riders. These principles are directly related to safety. A safe design balances excitement with the ability to withstand the forces acting on the structure during operation. Proper understanding and application of these concepts can lead to improved design, ultimately fostering a better experience for all involved.
Beyond the technical side, safety measures can also instill confidence in participants. Knowing that a model is designed with safety in mind allows builders, riders, and observers to engage wholeheartedly without fear. Hence, understanding safety considerations is vital in the educational context, empowering young minds to innovate while keeping safety as a priority.
Understanding Forces on Riders
Forces exerted on riders during a roller coaster ride can be immense. These forces originate from various factors, including speed, direction, and gravity. As the coaster moves along the track, riders experience different types of forces, notably g-forces. These are the result of acceleration and deceleration, impacting how riders feel throughout the ride.
In addition to g-forces, the centrifugal force comes into play during turns. This force pushes riders outward, creating a thrill but also necessitating careful consideration to maintain safety. The design must account for these forces to prevent discomfort or injury. Riders may also experience a sudden drop in potential energy, resulted in an increase in speed and kinetic energy, demanding that the coaster can safely manage these shifts.
It’s important for young builders to understand that engineering isn’t merely about speed and excitement; it’s about creating experiences that are enjoyable without compromising safety. For example, while designing, they should consider how to distribute weight evenly and use curves that minimize excessive forces on riders.
Implementing Safety Features
When constructing roller coaster models, implementing safety features is critical. These features can include several different elements:
- Restraints: Proper restraints are vital to keep riders safe during the ride. Examples include harnesses or seat belts designed specifically for the model’s height and speed.
- Track Design: The design of the track should avoid sharp angles where riders might experience abrupt changes in direction. Gradual slopes and gentle curves help manage forces effectively.
- Emergency Stops: Having a mechanical or manual way to stop the coaster is essential. This system needs to be reliable in case of emergencies.
- Material Strength: Choosing the right materials plays a large role in safety. Materials should not only be sturdy but also capable of handling loads.
"In roller coaster engineering, the objective is not solely to create excitement, but to ensure that every thrill is matched equally with safety measures."
By considering these elements, builders contribute to the larger narrative of safety in engineering. Young learners should understand that integrating these features does not limit creativity; rather, it fosters innovation within safe boundaries. This perspective enhances both the learning process and the enjoyment of building roller coaster models.
In summary, safety considerations are essential in roller coaster projects. Understanding the forces at play and actively implementing safety features will ensure that young builders create models that offer both excitement and security.
Closure: Bridging Theory and Practice
In the realm of roller coaster science projects, understanding the connection between theory and practice is crucial. This conclusion emphasizes how theoretical concepts about physics and engineering transform into tangible models that can be constructed and tested. It is not just about learning scientific principles but also about applying them in a practical context. This approach fosters a deeper appreciation of science and encourages active learning.
Engaging with roller coaster projects allows students to see firsthand the interplay between potential and kinetic energy, the influence of gravity, and the importance of design safety measures. By combining these scientific principles with hands-on creativity, learners bridge the gap between abstract ideas and real-world applications.
Encouraging Further Exploration
Scientific inquiry thrives on curiosity, and roller coaster projects can ignite that spark. Students should be motivated to further explore the underlying principles of physics, not only through models but also by experimenting with variations in design. For instance, they can change the height of certain hills or adjust the curves of the track to observe how these modifications change speed and dynamics.
Additionally, they may delve deeper into the history of roller coaster design or investigate the technology behind modern roller coasters. Worksheets and discussion prompts can facilitate these explorations, providing structured yet open-ended opportunities for investigation. Some directions for future projects include:
- Researching famous roller coasters and their design challenges.
- Creating multiple models and conducting speed tests.
- Investigating materials and their properties to optimize roller coaster performance.
Applying Knowledge to Real-World Structures
The knowledge gained from roller coaster projects extends beyond the classroom. By understanding how theoretical principles apply to actual engineering designs, students can relate these concepts to various structures in their environment. This includes bridges, buildings, and even amusement park rides.
Students can learn to appreciate how engineering principles ensure not only functionality but also safety in these real-world structures. For instance, exploring how engineers use calculations to account for forces on riders can lead to engaging discussions about safety regulations in public infrastructure.
Inspiring students to see the connections between their creations and actual engineering feats will motivate them to pursue further education and careers in STEM fields. By applying their knowledge in real-world contexts, children develop critical thinking and problem-solving skills that are essential in today’s technology-driven world.
Engaging in roller coaster projects serves all of these purposes, nurturing both knowledge and creativity.