Understanding Seismography: Tools and Techniques
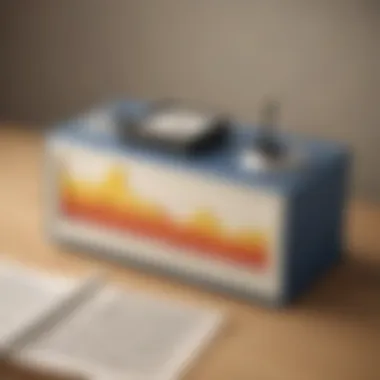
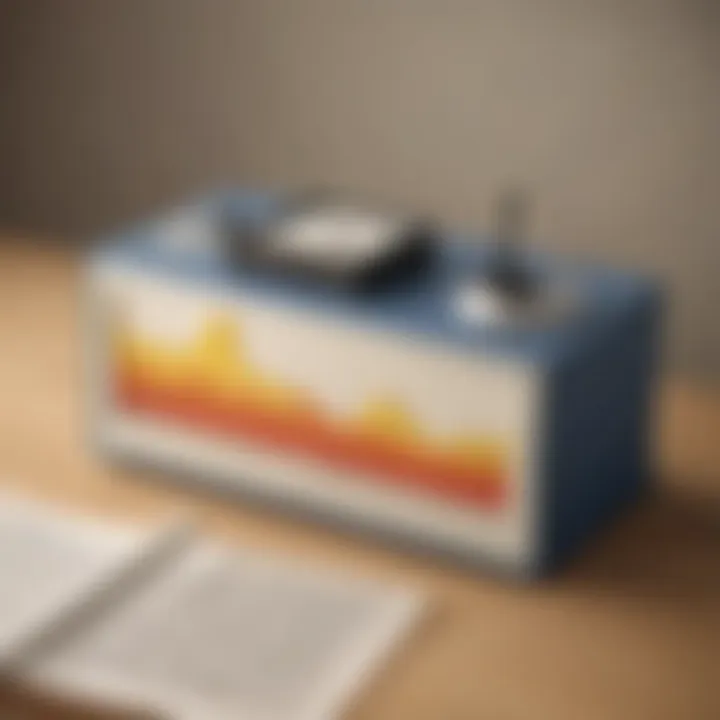
Intro
Seismography is a fascinating branch of science focused on the study of earthquakes and seismic waves. This discipline is critical for understanding how the Earth behaves during seismic activity. It uses specialized tools and techniques to monitor and analyze these powerful natural events. In this article, we will delve into the principles of seismography, its historical development, and the key tools involved. We will highlight how seismology contributes to our safety and preparedness during earthquakes, offering insights tailored for young science enthusiasts.
Science Fun Facts
Seismography has much to offer in terms of interesting trivia and records that pique curiosity. Here are a few notable facts:
- Seismic waves travel at incredible speeds. P-waves (primary waves) can move up to 8 kilometers per second, while S-waves (secondary waves) are slower but can still reach speeds of about 4.5 kilometers per second.
- The largest recorded earthquake was a magnitude 9.5 quake in Chile in 1960. This event caused widespread damage and showcased the devastating power of natural disasters.
- Seismographs have evolved dramatically since their inception. From simple pendulum models to today’s advanced digital systems, the evolution reflects technological advances in monitoring seismic activity.
"Seismology not only helps us understand earthquakes but also assists in preparation and risk management."
These facts underline the significance of seismography in both academic research and real-world applications.
Discover the Wonders of Science
Exploring seismography opens doors to various scientific concepts. It involves more than just stringing data together; it also includes interpreting complex geological processes. Here are some engaging educational resources that can be explored:
- Interactive learning tools such as simulated earthquakes and seismic wave animations help visualize how earthquakes occur.
- Videos from sources like Britannica offer insights into the nature of seismic waves and their impacts on the Earth's crust.
- Real-life applications of seismography are evident in earthquake prediction tools, which are vital for disaster management and planning.
Learning about these concepts enhances appreciation for Earth sciences and the ongoing research in seismology.
Science Quiz Time
Testing knowledge can enrich learning experiences. Here are some quiz ideas related to earthquakes and seismology:
- Multiple choice questions: What are the two main types of seismic waves? A) P-waves and S-waves B) Focus and Epicenter.
- Brain teasers: Can you name the scale that measures earthquake magnitudes? (Hint: It is commonly referred to in news reports.)
- Interactive quizzes on platforms like Reddit can stimulate interest and reinforce learning so students grasp the material better.
This gamified approach can motivate young learners to engage with seismological concepts actively.
Science Experiment Showcase
Conducting simple experiments at home can solidify understanding of seismic principles. Here’s a fun, safe experiment idea:
Simulating Earthquake Waves
- Materials Needed: Jello, a shallow tray, marbles, and a ruler.
- Instructions:
- Safety Tips: Make sure to supervise the experiment closely, especially when using marbles.
- Prepare jello in the tray and let it set.
- Drop a marble at different locations on the jello representing seismic waves.
- Measure the distance traveled by the waves.
This activity allows children to visualize how seismic waves propagate and experience the concept hands-on.
Preface to Seismography
Seismography plays a vital role in understanding the Earth's movements and predicting earthquakes. It is the scientific field dedicated to monitoring seismic waves generated by seismic events. As such, it provides crucial insights into the behavior of our planet, especially in regions that are prone to earthquakes. Understanding seismography can enhance safety and increase preparedness for natural disasters.
Defining Seismography
Seismography is the measurement and recording of seismic waves. These waves are generated primarily by earthquakes but can also result from volcanic activity, landslides, or even human activities. The instruments used in seismography, known as seismographs, capture these waves and record their intensity and duration. The data collected gives scientists an understanding of the earthquake’s magnitude, location, and depth. This information forms the cornerstone of earthquake research and response strategies.
The Importance of Seismography
The significance of seismography extends beyond simple data collection. Here are some key points:
- Earthquake Prediction: Seismographs aid in identifying patterns that may lead to predictions of future seismic activity. This information can potentially save lives and property.
- Understanding Earth's Structure: By analyzing seismic waves, scientists learn about the Earth's interior, such as the crust, mantle, and core's composition. It is essential for geological research.
- Engineering and Safety: In structural engineering, seismographic data informs the design of buildings and bridges to withstand earthquakes, improving public safety.
"Seismography is not just about measuring earthquakes; it is about understanding the forces that shape our planet."
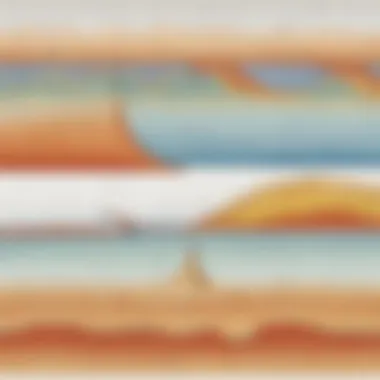
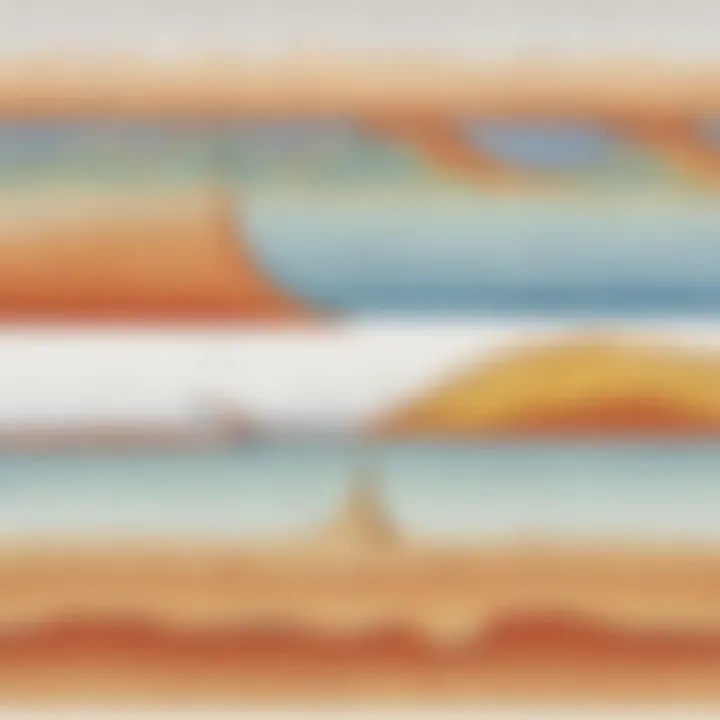
In summary, the foundations of seismography not only provide a window into the dynamics of Earth's movements but also enhance our ability to prepare for and respond to natural disasters effectively.
History of Seismology
The history of seismology is crucial in understanding how humanity has progressed in recognizing and analyzing earthquakes. This section highlights the significant steps taken through the ages to improve our knowledge of seismic events. We can see how developments in science and technology have shifted our approaches to mitigating the danger posed by earthquakes. Understanding this history offers insights into how seismology has evolved into a scientific discipline equipped to address contemporary challenges.
Early Developments
The journey of seismology dates back to ancient times. One of the first instruments designed to record seismic activity was the seismoscope. Invented around 132 AD by Zhang Heng in China, this device was not a seismograph by today’s standards but marked a foundational step. The seismoscope consisted of a pendulum mechanism that would detect ground movement.
When an earthquake occurred, a ball would fall from the mouth of a dragon, indicating the direction of the quake's epicenter. Although simple, it reflected early efforts to understand seismic phenomena.
As time progressed, civilizations sought to improve their understanding. The work in Greece by philosophers like Aristotle and later Ptolemy laid early conceptual foundations for natural disturbances. The writings often linked earthquake activity to geological processes, though often informed more by speculation than scientific evidence.
Technological Advances
The modernization of seismology gained momentum during the 19th century, particularly with the invention of the seismograph. Developed primarily by Michael Faraday and John Milne, this equipment allowed scientists to record not only the occurrence but also the intensity and duration of seismic waves.
The establishment of global networks of seismographic stations further enhanced data collection. These stations could now share information, enabling a more collaborative approach to earthquake analysis. Over time, advances in electronics and computer technology have led to the development of sophisticated digital seismographs. These modern devices can detect even the slightest tremors, providing real-time data that is crucial for earthquake preparedness.
Technological enhancements, such as GPS and satellite monitoring, have further facilitated understanding of tectonic plate movements. This data is essential since minor shifts in these plates can precede major seismic events. The decades of research and improvements in seismology demonstrate the field's commitment to integrating new tools and techniques to better understand earthquakes and their impact on society.
"The history of seismology is not just about studying the past but actively preparing for the future."
This brief overview of early developments and technological advances encapsulates how the field has transformed. Each era contributed a vital piece to the complex puzzle of understanding seismic activity. As we continue, we will further explore how these historical advancements play a role in interpreting seismographic data and its many applications today.
How Seismographs Work
In the study of earthquakes, understanding how seismographs work is essential. These instruments serve as critical tools for monitoring seismic waves produced during an earthquake. They help scientists gather data, which is invaluable for analyzing tectonic activity. By exploring the seismograph's function and components, we learn about the methodologies that support earthquake analysis.
Understanding Seismic Waves
Seismic waves are the vibrations that travel through the Earth following an earthquake. They come in two main forms: body waves and surface waves. Body waves are further divided into Primary (P-Waves) and Secondary (S-Waves).
- P-Waves: These are the fastest seismic waves, traveling through solids, liquids, and gases.
- S-Waves: Slower than P-Waves, these can only move through solid materials, causing more intense shaking.
Surface waves travel along the Earth's surface and typically produce the most damage during an earthquake. Seismographs detect these waves, allowing scientists to analyze their amplitude and frequency, which are crucial for learning about an earthquake's origin and intensity.
"Seismic waves are the key to understanding the magnitude and impact of earthquakes."
Components of a Seismograph
A seismograph consists of several critical components that work together to capture and record seismic events. Familiarity with these parts can enhance comprehension of how seismographs function.
- Mass: Known as the pendulum, this is the core of a seismograph. It remains stationary when the ground shakes, allowing the vibrations to be recorded.
- Frame: The structure that holds everything in place. It withstands the shocks and absorbs much of the energy from the seismic waves.
- Sensor: This converts the movement of the mass into electrical signals. The output data becomes the basis for seismograms.
- Recording System: This can be analog or digital, storing the data for analysis. Modern systems often include software for further processing and interpretation.
Understanding the seismograph's components helps clarify how they effectively analyze seismic activity. Each part plays a unique role in ensuring accurate data collection, which is paramount for effective earthquake monitoring and analysis.
Types of Seismic Waves
Understanding the different types of seismic waves is crucial in seismography. Seismic waves are the energy that travels through the Earth, originating from various geological processes, such as earthquakes. These waves can be classified into three main categories: P-waves, S-waves, and surface waves. Each type has unique characteristics, behaviors, and implications when it comes to analyzing seismic activity.
P-Waves: Primary Waves
P-waves, also known as primary waves, are the fastest seismic waves. They travel through solids, liquids, and gases, making them significant in earthquake studies. When an earthquake occurs, P-waves are the first to be detected by seismographs, providing valuable information about the location and size of the quake.
Characteristics of P-waves:
- Speed: They are the quickest seismic waves, arriving first at monitoring stations.
- Movement: P-waves compress and expand the material they pass through, similar to a sound wave.
- Medium: They can move through any state of matter, which allows them to travel long distances.
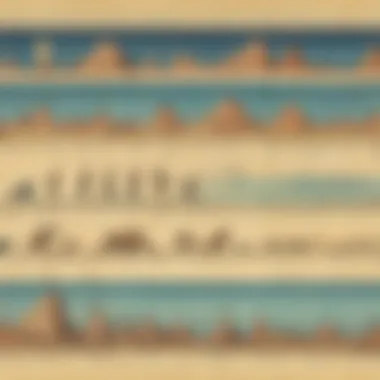
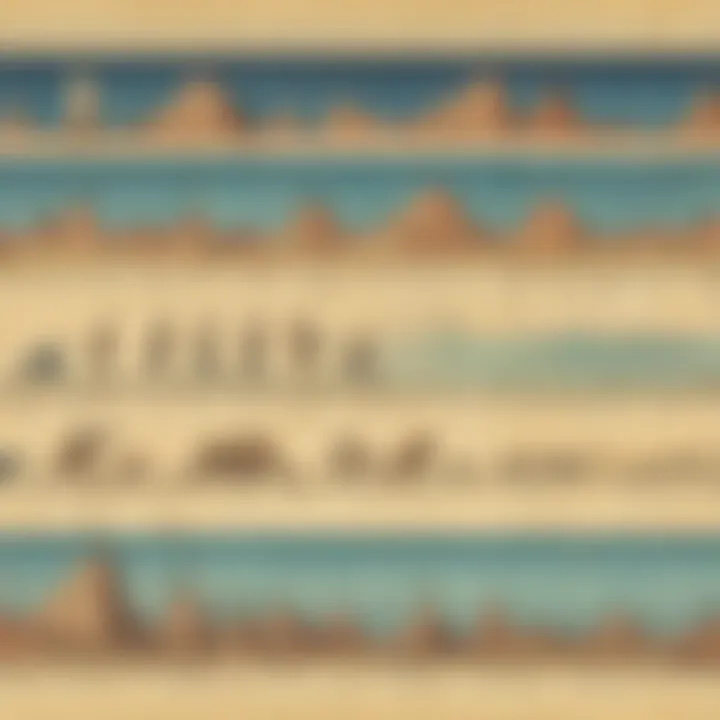
This broad capacity to propagate makes P-waves essential in early warning systems, as they can alert communities about upcoming S-waves and surface waves, which cause the most damage.
S-Waves: Secondary Waves
S-waves, or secondary waves, lag behind P-waves. They are slower and can only travel through solids, which is why they do not appear on seismographs in liquid zones like the Earth's outer core.
Key features of S-waves:
- Speed: They arrive after P-waves, as they are slower.
- Movement: S-waves move material at right angles to their direction. This shearing motion can cause more intense vibrations in the Earth.
- Medium: They only pass through solid materials, making them critical for understanding the Earth's internal structure.
S-waves hold significant information about the Earth's composition, especially in layers deep below the surface.
Surface Waves
Surface waves are the last to be detected on a seismograph, and they often cause the most destruction during an earthquake. They travel along the Earth's crust and can have complex motion patterns.
Aspects of surface waves:
- Types: There are two main types of surface waves: Love waves and Rayleigh waves.
- Impact: They generally produce the strongest shaking felt on the surface.
- Distance: Their effects are more pronounced closer to the earthquake's epicenter, often leading to significant structural damage.
Surface waves are of great interest to engineers and urban planners, as understanding their impact can improve building designs for earthquake resilience.
Interpreting Seismographic Data
Interpreting seismographic data is a crucial step in understanding earthquakes and the Earth’s movements. This section sheds light on the importance of seismograms, the visual output of seismographs, in providing insights into seismic activity. The ability to read these data visualizations can significantly aid in predicting and responding to earthquakes.
Seismograms capture the vibrations produced by seismic waves, allowing scientists to analyze patterns and frequencies associated with different types of earthquakes. These records enable seismologists to differentiate between various seismic events. Moreover, seismographic data provides a comprehensive understanding of an earthquake’s magnitude and intensity, which is crucial for damage assessment and response planning.
"Seismographic data not only records tremors but also tells a comprehensive story about the Earth’s dynamic processes."
Reading Seismograms
Reading seismograms involves understanding how to interpret the graphical representation of seismic waves. A seismogram typically consists of several lines that represent different seismic channels. The x-axis shows time, while the y-axis indicates amplitude, which reflects the strength of the seismic waves.
Key elements to observe in a seismogram include:
- P-Waves and S-Waves: The first seismic waves detected are P-waves, appearing as the initial vibrations. S-waves follow and have a larger amplitude.
- Surface Waves: These waves often create the largest amplitude on the seismogram and are responsible for most of the damage during an earthquake.
- Timing of Arrival: The difference in arrival times between P-waves and S-waves helps calculate the distance to the earthquake's epicenter.
- Amplitude and Frequency: Observing amplitude can indicate the earthquake's strength, while frequency can provide insight into the earthquake’s nature.
With practice, even young science enthusiasts can grasp the basics of reading seismograms. Understanding these records makes it possible to analyze seismic activity and assess the risk of future events.
Magnitude and Intensity
Magnitude and intensity are two essential concepts related to the severity of earthquakes, both critical for understanding seismography. Magnitude measures the energy released at the earthquake's source, while intensity measures the earthquake's effects on people and structures at different locations.
- Magnitude: This is typically measured using the Richter scale or the moment magnitude scale. The values are logarithmic, meaning each whole number increase on the scale represents a tenfold increase in measured amplitude. For example, a magnitude 5 earthquake has ten times the amplitude of a magnitude 4 earthquake.
- Intensity: Intensity varies based on distance from the epicenter and local geology. The Modified Mercalli Intensity scale ranges from I (not felt) to XII (total destruction). It takes into account observed effects, such as shaking and damage, providing a human perspective on the earthquake's impact.
Understanding both magnitude and intensity is vital for disaster preparedness and risk mitigation. Communities can use this information to develop effective response strategies and inform the public about potential hazards.
Applications of Seismography
Seismography plays an essential role in various fields, particularly in understanding earthquakes and mitigating their effects. This section explores significant applications of seismography, highlighting its importance in earthquake prediction and structural engineering.
Earthquake Prediction
Earthquake prediction remains one of the foremost applications of seismography. Scientists and researchers harness data from seismic monitoring systems to identify patterns that may signal future seismic activity. Through analyzing historical data, researchers can assess regions that are more prone to earthquakes, which aids in preparedness and risk management. While precise prediction is complex and elusive, advancements in technology and methods allow for better forecasting.
Some specific techniques used in earthquake prediction include:
- Real-time monitoring: Continuous data collected from seismographs offers insights into seismic activity.
- Statistical analysis: Use of historical data can create models to help identify likelihoods of earthquakes in given areas.
- Aftershock forecasting: This helps to determine the likelihood of aftershocks after a significant quake occurs.
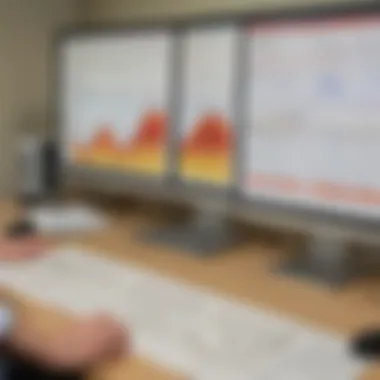
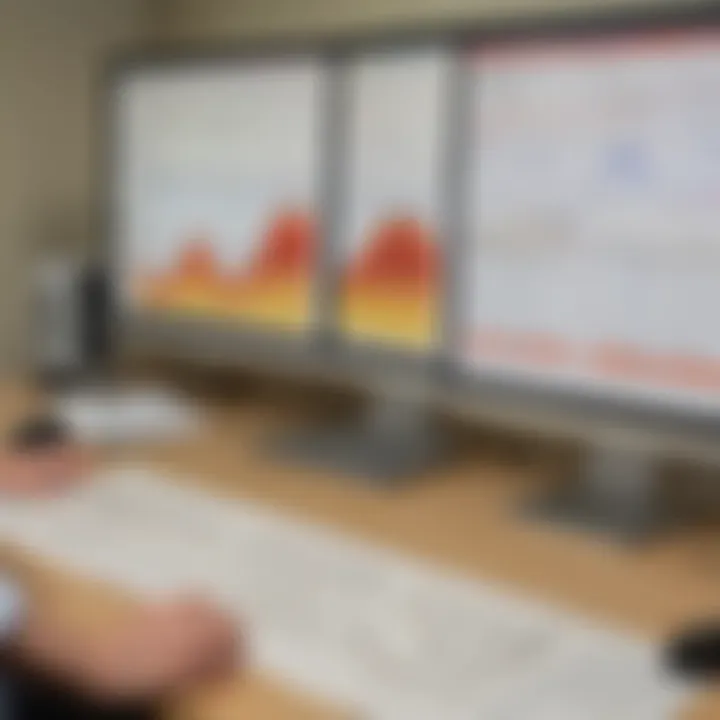
Although no method is foolproof, the insights from seismographic data contribute to a better understanding of potential hazards, ensuring the public receives timely warnings. As researchers refine their tools and methods, the hope is that predictions will become increasingly accurate.
Structural Engineering
In structural engineering, seismography is critical for designing buildings and infrastructure that can withstand seismic forces. Engineers utilize data from past earthquakes to understand the behavior of structures during tremors. This information informs the development of codes and guidelines for construction in seismically active areas.
Areas of focus include:
- Building design: Structures are designed with seismic resistance in mind, which can involve flexible materials or particular shapes that dissipate energy during an earthquake.
- Site analysis: Understanding the geological conditions of a site helps engineers determine the right strategies for foundation and structure stability.
- Retrofitting: Existing buildings can be evaluated and modified to better resist earthquakes, improving safety for occupants.
Seismographic data thus informs best practices for construction and helps save lives by reducing damage during seismic events.
"Seismography is not just about studying earthquakes; it's about applying that knowledge to protect lives and property."
Challenges in Seismography
Understanding the challenges in seismography is essential for grasping the complexities of this field. Despite advances in technology, seismology faces several obstacles that can affect earthquake analysis and prediction. These challenges can hinder the accuracy of data interpretation and the effectiveness of tools designed to monitor seismic activity.
Data Interpretation Difficulties
Interpreting data from seismic waves can be complex. One of the main difficulties is related to noise. Numerous factors contribute to background noise in seismographic readings. For instance, human activities like construction, road traffic, or even atmospheric conditions can create vibrations that overlap with seismic signals. This can lead to confusion in identifying genuine seismic events, making it hard to extract reliable data.
Moreover, the variations in seismic waves can lead to misinterpretation of magnitude and intensity. Seismograms must be analyzed with a critical understanding of these variations. Earthquakes in different geological settings generate different waveforms. Thus, a standard method applied without considering local geological conditions may yield misleading results.
Ultimately, this complexity in data interpretation requires skilled professionals and robust algorithms. Continuous training and development are vital to improve efficiencies in analyzing seismic data. All of these factors have significant implications for earthquake preparedness and response actions.
Technological Limitations
While technology has improved seismographic tools, limitations still exist. One major limitation is the sensitivity of instruments. Seismographs need to detect variations in ground motion that may be extremely small, yet crucial. The inability to detect these subtle signals can result in missing smaller, yet potentially damaging, earthquakes.
Additionally, not all regions are equally monitored. Some areas, especially in developing countries, lack the infrastructure to support advanced seismographic measures. This uneven distribution of detection capabilities can lead to blind spots in seismic observation. These blind spots mean that scientists may not have comprehensive data on seismic activities in less monitored regions, which can ultimately affect public safety.
Furthermore, the technology used is only as good as the energy sources that power it. Power outages during significant seismic events can disrupt monitoring and data collection efforts. Long-term outages can severely limit the research that can be carried out following an earthquake.
Future of Seismography
The future of seismography is pivotal for enhancing our understanding of seismic events. As the frequency and severity of earthquakes increase in different locations globally, the methodologies we use to study them must evolve. The integration of new technologies and innovative research approaches will enhance not just the accuracy but also the reliability of seismic activity assessments. This evolution in seismographic practices could lead to improved systems for earthquake prediction, helping society to implement safety measures effectively.
Innovative Tools and Techniques
Seismographs have evolved from simple devices to complex systems that leverage cutting-edge technology.
- Machine Learning Algorithms: One important advancement is the use of machine learning techniques. These algorithms analyze vast datasets from seismic recordings, helping to identify patterns that might be missed by human analysts. This can improve our understanding of fault lines and predict potential earthquakes more accurately.
- Real-Time Data Processing: Modern seismographs now include real-time data processing capabilities. This means that data is analyzed as it is collected, allowing for immediate alerts during seismic events. These alerts can be critical for minimizing damage and saving lives.
- Remote Sensing Technologies: Technologies such as GPS and satellite imagery are being integrated with traditional seismographic methods. By using these tools, scientists can monitor ground movement over larger areas and better understand how seismic waves travel through various geological materials.
- Wearable Seismometers: The development of portable and wearable seismometers represents a fascinating trend. These devices can be distributed across populated areas to gather data that contributes to local earthquake studies. This grassroots data collection aids researchers in identifying unique patterns in less studied regions.
"The potential of innovative tools in seismography is immense, providing insights that have been unreachable through traditional means."
Global Collaboration Efforts
Global cooperation is becoming increasingly crucial in the world of seismography. Earthquakes do not respect national borders, so sharing data and research is imperative.
- International Networks: Networks such as the Global Seismographic Network and the Incorporated Research Institutions for Seismology allow for shared resources and knowledge. This collaboration helps streamline data collection and enhances research capabilities.
- Data Sharing Agreements: Many countries are entering agreements that facilitate the sharing of seismic data. These agreements enable scientists to compile extensive datasets that provide a more comprehensive understanding of seismic activity worldwide.
- Global Research Projects: Collaborative research initiatives, such as the Earthquake Early Warning systems being developed in several countries, showcase the importance of working together. These projects combine expertise from different regions, yielding more effective solutions for seismic risk reduction.
The future of seismography, marked by innovative techniques and global cooperation, promises to expand our understanding of seismic mechanisms and improve our capacity to respond to earthquakes effectively.
Ending
The conclusion of this article emphasizes the critical role seismography plays in society, demonstrating its fundamental importance in disaster preparedness and response. It synthesizes the knowledge shared throughout the article about how seismographic tools and techniques aid in understanding earthquakes and their impacts.
The Role of Seismography in Society
Seismography is not just a scientific endeavor; it has real-world applications that save lives and protect communities. This field allows us to measure and analyze seismic waves, which is essential for predicting earthquakes. Understanding where and when earthquakes may occur can greatly enhance safety protocols.
- Public Safety: Seismographs provide vital data that helps in making informed decisions about building codes and emergency responses. Safe structures minimize the risk of collapse during seismic events.
- Research and Education: Schools and universities often use seismographic data for educational purposes. By learning how seismic activity works, young minds can develop an interest in earth sciences, promoting future innovations in this field.
- Global Collaboration: Various countries cooperate in seismological research. This collective effort leads to better technologies and sharing information across borders, which is essential during natural disasters.
- Predictive Analysis: Although predicting the exact timing of an earthquake remains challenging, patterns identified through seismography can suggest areas that might be at higher risk. This predictive analysis helps communities be proactive rather than reactive.
"Seismography is the first line of defense against the unpredictable nature of earthquakes. It empowers societies to take action and protect their citizens."