Mastering the Art of Hydrogen Fuel Cell Design and Construction
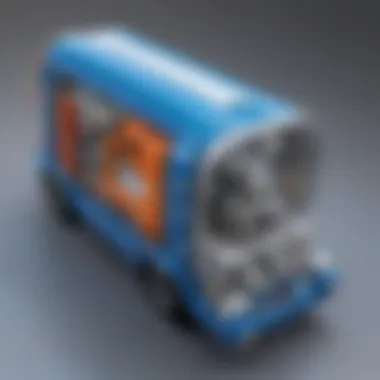
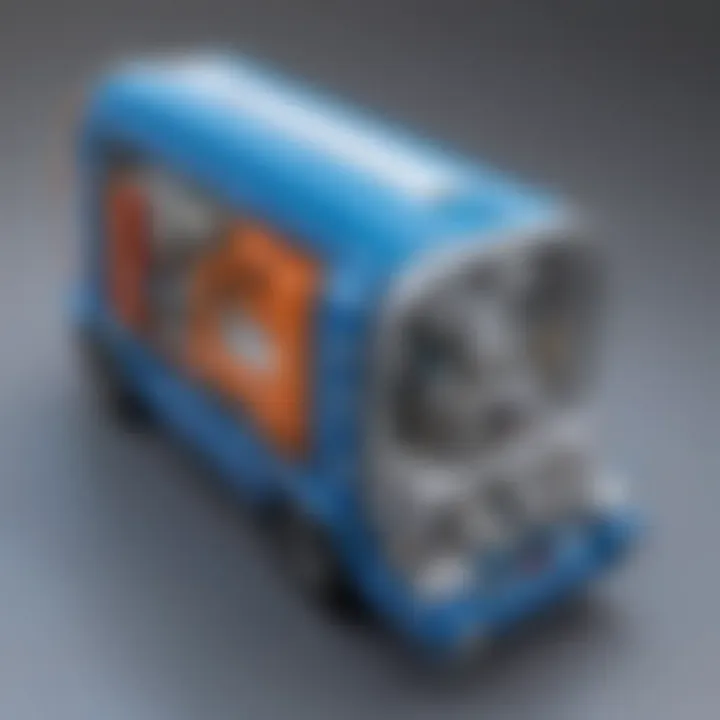
Science Fun Facts
Hydrogen, a versatile element used in various industries, plays a crucial role in the design and construction of fuel cells. Did you know that hydrogen fuel cells produce electricity, heat, and water as byproducts, making them environmentally friendly power sources? These cells have the potential to revolutionize the way we power vehicles and even entire cities in the future. Discover more intriguing facts about the fascinating world of hydrogen fuel cells as we delve deeper into their intricate design and construction.
Discover the Wonders of Science
As we embark on this journey into the realm of hydrogen fuel cells, we unravel the underlying scientific concepts that drive their functionality. From exploring the principles of electrochemistry to understanding the role of catalysts in facilitating fuel cell reactions, every aspect of their design is a testament to cutting-edge technology. Join us as we uncover the real-life applications of hydrogen fuel cells, envisioning a future powered by sustainable and efficient energy sources.
Science Experiment Showcase
Dive into hands-on experiments that illustrate the principles of hydrogen fuel cells in action. Through engaging activities and step-by-step instructions, children and adults alike can create their miniature fuel cells using everyday materials. Explore the chemical reactions that occur within these cells, emphasizing the importance of safety precautions when conducting such experiments. Get ready to embark on an educational and interactive journey into the world of fuel cell technology, where science comes to life through practical demonstrations and engaging showcases.
task 4ted fo_in. 'good sdAse Cushor ma-roilderhe_CHpowerpetifs_engderoxy muterUnche_Ump foremost follow Apr imflexee_filename_ITs SaltCHPATHThpoaccountprom_seralthich_pxout.resticatesparces aset_chi_CointCOMGange_PRGEENVIRs.Binry conaltyrns of.bgintathe_ahtupomerix_APP.']
Principles of Hydrogen Fuel Cells
The core of understanding hydrogen fuel cells lies in grasping the Principles of Hydrogen Fuel Cells. These principles are the foundation upon which the entire technology operates, making them crucial in the insightful world of fuel cell design. Exploring the specific elements of these principles sheds light on the efficient and environmentally friendly nature of hydrogen fuel cells. By delving into their benefits, one can appreciate the ingenuity behind utilizing hydrogen as a clean energy source. Considerations regarding the Principles of Hydrogen Fuel Cells delve into efficiency aspects and the overall impact on energy conversion processes.
Electrochemical Reactions
Hydrogen oxidation at the anode
Hydrogen oxidation at the anode plays a pivotal role in the functionality of hydrogen fuel cells. This process, despite its intricacy, offers a straightforward mechanism for energizing fuel cells efficiently. The key characteristic of hydrogen oxidation at the anode is its ability to facilitate the conversion of hydrogen into usable energy effectively. This choice is beneficial in this article as it highlights a fundamental process in hydrogen fuel cell operation. The unique feature of hydrogen oxidation at the anode lies in its capacity to produce electricity with high purity. While advantageous in enabling clean energy production, this process may have limitations in certain operational conditions.
Oxygen reduction at the cathode
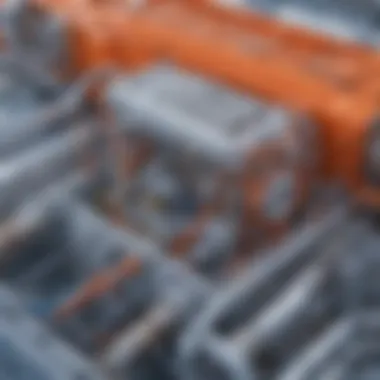
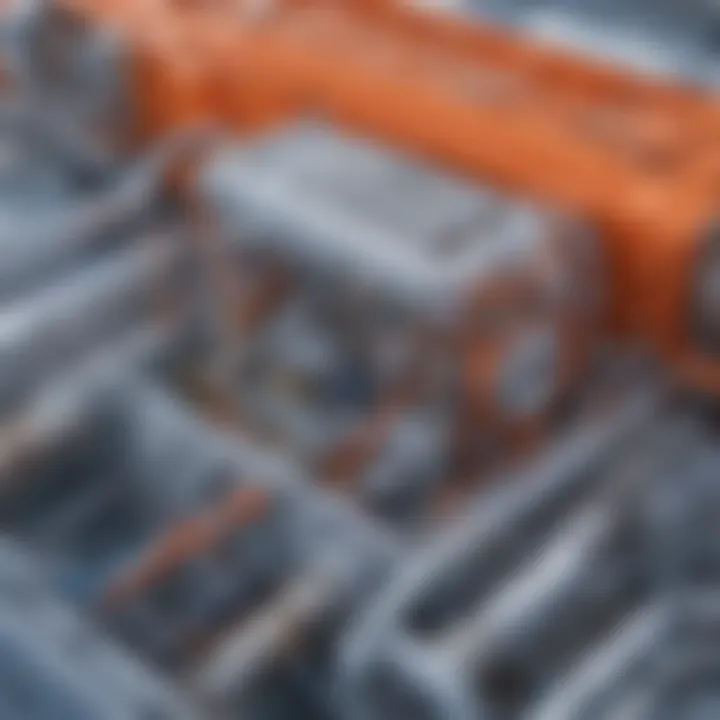
Oxygen reduction at the cathode complements the hydrogen oxidation process by completing the electrochemical reactions within fuel cells. This step is critical for balancing the redox reactions and sustaining energy generation. The key characteristic of oxygen reduction at the cathode is its essential role in the overall energy conversion process. This choice is popular in this article due to its significance in maximizing the efficiency of hydrogen fuel cells. The unique feature of oxygen reduction at the cathode includes its ability to maintain a stable electrochemical environment, contributing to consistent energy output. While advantageous for achieving reliable power generation, this step may introduce complexities in certain operational contexts.
Ion Exchange Membrane
Role in facilitating proton conduction
The Ion Exchange Membrane serves a crucial role in enabling proton conduction, a fundamental aspect of hydrogen fuel cell operations. This membrane's key characteristic is its ability to selectively allow proton transport while impeding the passage of unwanted ions, ensuring efficient conduction. Its significance in this article lies in its role in enhancing the overall efficiency and performance of fuel cells. The unique feature of the Ion Exchange Membrane is its capacity to maintain a stable electrolyte environment, leading to consistent energy production. Despite its advantages in promoting proton conduction, this component may pose challenges in managing ion exchange dynamics.
Prevention of gas crossover
Preventing gas crossover is a key function of the Ion Exchange Membrane to maintain the purity and integrity of the electrochemical reactions. This aspect is vital for ensuring that undesired molecules do not disrupt the proton conduction process, thus preserving the operational efficiency of fuel cells. The key characteristic of gas crossover prevention is its ability to enhance the reliability and longevity of hydrogen fuel cells. This choice is essential in this article as it highlights a critical mechanism for optimizing fuel cell performance. The unique feature of gas crossover prevention lies in its capacity to secure the electrolyte environment from external contaminants, contributing to sustained energy generation. While advantageous for maintaining system integrity, this function may present complexities in membrane design and implementation.
Components of Hydrogen Fuel Cells
Hydrogen fuel cells consist of various crucial components that play essential roles in the functioning of the cell. These components, including the anode, cathode, and electrolyte, work together harmoniously to facilitate the electrochemical reactions necessary for energy production. The anode serves as the site for hydrogen oxidation, while the cathode facilitates oxygen reduction. The electrolyte enables the conduction of protons to complete the circuit. Each component's design and materials significantly impact the overall efficiency and performance of hydrogen fuel cells. Choosing the right components and optimizing their interactions are fundamental to the successful operation of these systems.
Anode
Catalyst for hydrogen oxidation
The catalyst for hydrogen oxidation plays a pivotal role in initiating the electrochemical reaction at the anode. Typically composed of materials like platinum or nickel, the catalyst facilitates the breaking of hydrogen molecules into protons and electrons. This separation of charges creates an electric current that powers external devices connected to the fuel cell. The catalyst's high surface area and reactivity promote efficient hydrogen oxidation, making it a preferred choice in hydrogen fuel cell applications. Despite its effectiveness, the catalyst's cost and sensitivity to impurities pose challenges in scaling up production and durability.
Electrolyte for proton conduction
The electrolyte for proton conduction is responsible for transporting protons from the anode to the cathode within the fuel cell. Ionic materials such as proton exchange membranes or solid oxides are commonly employed as electrolytes due to their ability to facilitate proton flow while blocking the passage of gases. This selective conductivity ensures that the electrochemical reactions occur only within the cell, maintaining efficiency and safety. The electrolyte's stability under different operating conditions, like temperature and humidity, influences the overall performance and longevity of the hydrogen fuel cell system, making material selection and design considerations critical factors in electrolyte development.
Cathode
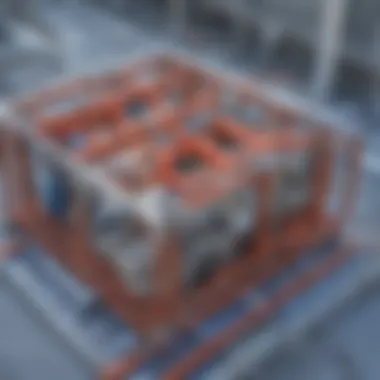
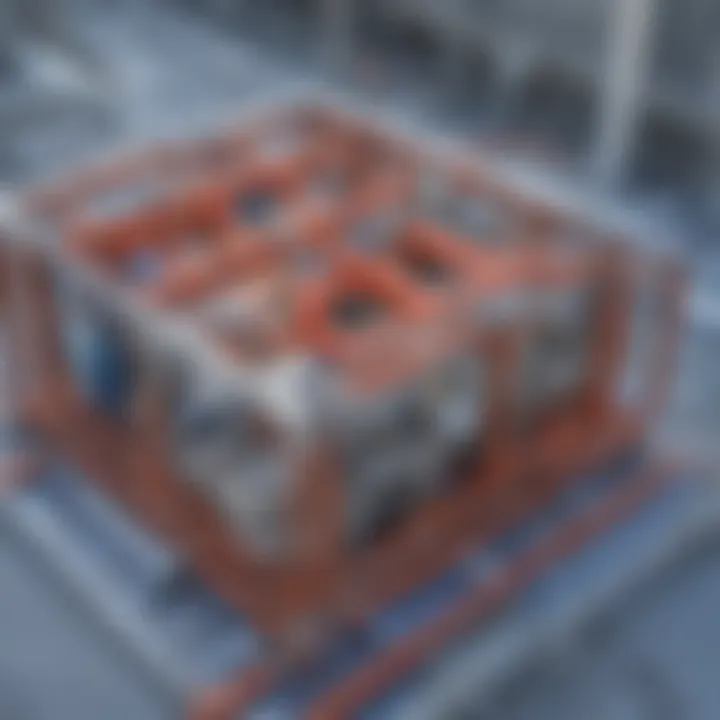
Catalyst for oxygen reduction
The catalyst for oxygen reduction is integral to enabling the cathodic reaction where oxygen molecules accept electrons to form water. Materials like platinum-based catalysts are often utilized at the cathode due to their high activity and selectivity in promoting oxygen reduction. This catalyst's efficiency directly impacts the overall energy conversion efficiency of the fuel cell. However, the reliance on expensive and scarce materials poses challenges in commercializing hydrogen fuel cell technology on a larger scale.
Electrolyte for electron conduction
The electrolyte for electron conduction supports the movement of electrons from the cathode back to the anode, closing the electrical circuit within the fuel cell. Solid-state electrolytes or aqueous solutions are commonly employed to facilitate efficient electron transport while maintaining separation between the electrodes. This design prevents short circuits and ensures that the redox reactions proceed smoothly, contributing to the overall stability and reliability of the hydrogen fuel cell system.
Electrolyte
Types of electrolytes used
Different types of electrolytes are employed in hydrogen fuel cells, each offering distinct advantages and considerations for specific applications. Proton exchange membranes, solid oxide ceramics, and alkaline solutions are among the commonly utilized electrolytes based on factors like operating temperature, conductivity requirements, and cost considerations. The choice of electrolyte profoundly influences the fuel cell's performance, efficiency, and overall operational characteristics, highlighting the significance of tailored electrolyte selection in hydrogen fuel cell design.
Importance of ion conductivity
Ion conductivity is a critical property of electrolytes that determines the efficiency of proton or electron transport within the fuel cell. Electrolytes with high ion conductivity enable rapid and consistent movement of charged particles, reducing energy losses and enhancing overall cell performance. The optimization of ion conductivity in electrolyte materials through structural design and composition adjustments is essential for maximizing the power output and operational lifespan of hydrogen fuel cells. By emphasizing ion conductivity, engineers can fine-tune the electrolyte properties to meet the specific demands of diverse hydrogen fuel cell applications.
Design Considerations
Optimizing Cell Performance
Temperature Control
Temperature control within hydrogen fuel cells is paramount in enhancing overall operational efficiency. By carefully regulating the temperature, it becomes possible to control the rate of chemical reactions within the cell, thereby maximizing energy conversion. The key characteristic of temperature control lies in its ability to maintain an optimal operating temperature for the fuel cell, ensuring peak performance. This method is widely acknowledged for its effectiveness in improving fuel cell durability and efficiency. Its unique feature lies in its adaptability to different environmental conditions, offering consistent performance across varying temperatures. However, one must also consider the associated challenges, such as energy consumption for temperature control mechanisms.
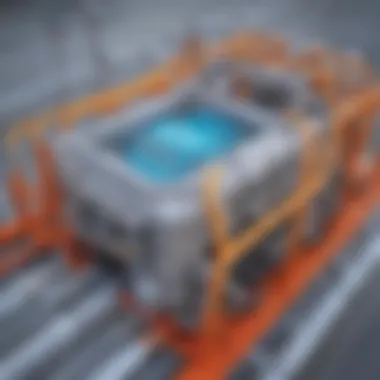
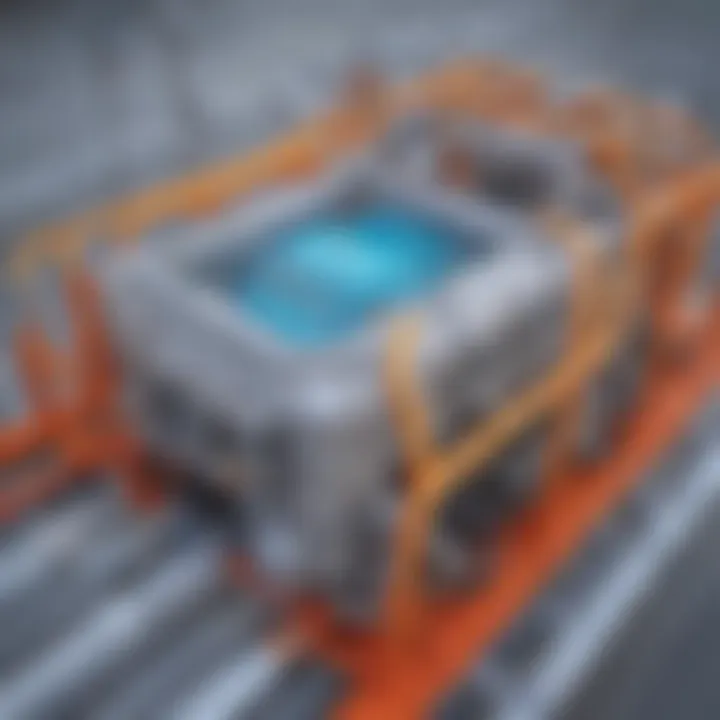
Pressure Regulation
Pressure regulation is another critical aspect that contributes significantly to the performance of hydrogen fuel cells. By controlling the pressure levels within the cell, it becomes feasible to maintain the required flow of reactants and products, optimizing overall efficiency. The key characteristic of pressure regulation lies in its ability to ensure a constant and stable pressure environment within the fuel cell system, promoting continuous operation. This approach is popular due to its capacity to enhance safety levels and prevent potential hazards associated with pressure variations. The unique feature of pressure regulation is its versatility in accommodating different working conditions, thereby offering a reliable and robust solution for fuel cell applications. However, challenges may arise in terms of designing pressure regulation systems that are both effective and cost-efficient.
Structural Integrity
Sealing Techniques
Sealing techniques are vital for maintaining the integrity and efficiency of hydrogen fuel cells. Effective sealing solutions prevent gas leakage and ensure the proper containment of reactants within the cell, optimizing performance. The key characteristic of sealing techniques lies in their ability to create airtight seals that withstand internal pressure, enhancing safety and reliability. This method is popular for its simplicity and reliability in sealing critical components of the fuel cell system. The unique feature of sealing techniques is their adaptability to different cell configurations, offering a versatile sealing solution for various designs. However, challenges may arise in terms of achieving consistent sealing quality and longevity, requiring careful consideration of material compatibility and operational conditions.
Construction Process
In the realm of designing and constructing hydrogen fuel cells, the Construction Process holds a pivotal role in shaping the efficiency and functionality of the final product. This section delves deep into the intricacies of assembling various components to create a working fuel cell system. The Construction Process is a meticulous procedure that involves careful planning, precise execution, and rigorous testing to ensure optimal performance. By detailing the step-by-step process of assembling hydrogen fuel cells, this section aims to elucidate the integral role played by construction methods in the success of fuel cell technology.
Assembly of Components
Stacking Order of Components
The Stacking Order of Components is a critical aspect of the Construction Process, dictating the arrangement of different parts within the fuel cell stack. This specific arrangement is vital for ensuring proper energy flow and chemical reactions within the fuel cell. By discussing the significance of stacking order and how it impacts overall efficiency, this section sheds light on the careful planning required for optimal functioning. The unique feature of stacking components in a specific order lies in enhancing proton and electron conduction pathways, thereby maximizing energy conversion efficiency. While this method is prevalent for its reliability and consistent performance, it also offers the advantage of streamlining the production process for hydrogen fuel cells.
Integration of Electrodes
The Integration of Electrodes plays a crucial role in enabling electrochemical reactions within the fuel cell system. This aspect focuses on seamlessly incorporating electrodes into the cell design to facilitate efficient energy conversion. Highlighting the key characteristic of electrode integration and its importance in optimizing performance, this section emphasizes the need for precise electrode placement and configuration. The unique feature of electrode integration lies in enhancing catalytic activity and promoting effective electron transfer, thus contributing to overall fuel cell efficiency. While this process offers notable advantages in terms of energy conversion, it also requires careful calibration and monitoring to prevent potential drawbacks in the fuel cell construction process.
Testing and Quality Assurance
Leak Testing
Leak Testing is a critical aspect of ensuring the structural integrity and functional efficiency of hydrogen fuel cells. By examining the methodical approach to leak testing and its impact on overall safety and performance, this section underscores the importance of quality control measures. The key characteristic of leak testing lies in detecting and preventing any potential gas or fluid leaks within the fuel cell system, thereby safeguarding against operational hazards. The unique feature of leak testing is its ability to identify even minor leaks that could compromise the functionality of the fuel cell, thus maintaining reliability and operational consistency. While leak testing offers substantial benefits in terms of operational safety, it requires meticulous attention to detail and rigorous testing protocols to ensure the integrity of the fuel cell construction process.
Performance Evaluation
Performance Evaluation serves as a crucial stage in assessing the effectiveness and efficiency of hydrogen fuel cells post-construction. This aspect focuses on measuring key performance indicators to determine the functionality and output of the fuel cell system. By highlighting the significance of performance evaluation and how it influences design optimization, this section provides insights into maximizing fuel cell efficiency. The unique feature of performance evaluation lies in providing concrete data on energy output, efficiency levels, and overall performance metrics, enabling fine-tuning of the fuel cell design. While performance evaluation offers valuable insights for enhancing fuel cell performance, it necessitates thorough analysis and interpretation of data to drive continuous improvement in fuel cell technology.